Biosynthesis of DLLAE blended silver nanoparticles and their response against periodontitis triggering bacteria
Abstract
Facts over microorganisms to predominate periodontitis, shifting of human microbiota by Dimocarpus longan (D. longan) plant, and potentiation of antimicrobial activity by biosynthetic silver nanoparticles (SNPs) intended present study to biosynthesize, optimize, characterize and evaluate the antimicrobial potential of silver nanoparticles (SNPs) obtained using D. longan leaves aqueous extract (DLLAE). Study involved preparation of DLLAE using decoction method. The DLLAE was subjected to biosynthesis of SNPs followed by optimization (using UV-Visible spectrometry), characterization (by FTIR, FESEM, XRD, and EDX), stability, and antimicrobial activity of SNPs against periodontitis triggering human microflora. Biosynthesized SNPs exhibited signal between 416-453 nm. Optimization study established AgNO3 concentration (5 mM), pH 4, DLLAE and AgNO3 ratio (1:9) and temperature (60°C) as parametric requirement for SNPs biosynthesis using DLLAE. Stability study exhibited signal between 489-553 nm supporting SNPs stability. Characterization data of FESEM showed that SNPs were poly dispersed, and spherical shaped. Biosynthesized SNPs size ranged from 74.82 nm to 131.5 nm. The XRD data revealed presence of signals at 38.08°, 44.33°, 64.47°, and 78.83° 2θ values indexed to silver cubic structure planes. In EDX study, silver exhibited strong signal (55.54%). Antimicrobial investigation explored the high inhibitory potential of SNPs against B. subtilis and P. aeruginosa and low inhibitory potential against S. aureus and E. coli. Present study conclude that biosynthesis of SNPs using DLLAE is an efficient method and biosynthetic SNPs possess high antimicrobial potential against P. aeruginosa and B. subtilis the periodontitis triggering pathogens.
Keywords
SNPs, D. Longan, Optimization, Characterization, Antimicrobial
Introduction
Current decade reports humans to own 1:1 bacteria and cells. A small disturbance in human microbiota manifests in several infectious diseases (Sender, Fuchs, & Milo, 2016). Among all infectious disease periodontitis is claimed to be most complicated microbial infection. Periodontitis is connected with dental biofilm dysbiosis, which results in chronic inflammation of soft tissue periodontal lining, and tooth/alveolar bone destruction (Lourenςo, Spencer, Alm, & Colombo, 2018). Periodontitis manifests in progression of cancer, cardiovascular diseases, obesity, respiratory disease, rheumatoid arthritis, and diabetes. Facts suggest Pseudomonas aeruginosa (P. aeruginosa), Staphylococcus aureus (S. aureus), Escherichia coli (E. coli), and Bacillus subtilis (B. subtilis) to trigger periodontitis. Several conventional antimicrobials used in periodontitis infections offers adverse effects like multiple drug resistance, immune suppression, allergic reactions, and high mortality risk. Thus, there is a necessity for natural alternatives (Fuloria, Fuloria, Chia, Karupiah, & Sathasivam, 2019).
Current decade witnesses enormous research over SNPs ability to augment the antimicrobials and other biomedicines activity. The inherent properties (like: high antibiotic potential, increased shelf life, low toxicity, cost-effectiveness, and easy availability of herbal drugs) always challenges the researchers to investigate drugs for treatment of periodontal infections using plants source (Fuloria et al., 2019). Formulation of SNPs using D. longan seeds extracts (bearing high antimicrobial activity) attracts the investigators (Phongtongpasuk, Poadang, & Yongvanich, 2017), but formulation of SNPs using D. longan leaves is yet an area to be explored. Based on the evidences, current study was planned to biosynthesize CSNPS using DLLAE and evaluate against periodontitis triggering bacteria.
Materials and Methods
The chemicals like: potassium bromide (KBr), silver nitrate (AgNO3), sodium hydroxide (NaOH), calcium chloride (CaCl2), sodium oxalate, sodium chloride, Tris buffer, dimethyl sulfoxide (DMSO), and nutrient agar were procured from Fisher chemicals, Sigma Aldrich, SD Fine, and Hi-Media. The SNPs were biosynthesized using Dimocarpus longan leaves aqueous extract (DLLAE). The glass wares were cleaned using (deionized water), dried (at 160°C) and plastic ware were autoclaved before initiation of antimicrobial experiment.
DLLAE preparation
The experimental procedure for DLLAE preparation was based on reported method with slight modification (Cecilia et al., 2016). Briefly, the fresh D. longan leaves were collected (from Kampung Baru, Bedong, Kedah, Malaysia), washed with water (for impurities elimination), dried (40-50oC), and powdered (using blender) for further investigation. The D. longan leaves aqueous extract (DLLAE) was prepared by boiling mixture of blended D. longan leaves powder (25 g), de-ionised water (95 ml) and ethanol (5 ml). Mixture was allowed to cool and filtered using 4-fold muslin cloth and Whatman no. 1 filter paper to offer pure DLLAE. The DLLAE which was stored at 8-10°C in a refrigerator subjected to further investigations and SNPs synthesis.
SNPs biosynthesis
The biosynthesis of SNPs was conducted according to the reported procedure with slight modification (Fuloria et al., 2019; Fuloria et al., 2019). Briefly, the SNPs biosynthesis was done by mixing 1 ml of D. longan extract with 9 ml of AgNO3 solution (5 mM). The mixture was left for incubation for 24 h in a dark cardboard to undergo reduction. The AgNO3 solution after reduction (change in color to brown), was subjected to centrifugation for 90 minutes at 5000 rpm.
Supernatant layer was removed off to yield SNPs. The SNPs were further rewashed with deionized water, re-centrifuged (using the same parameters) and air dried to yield pure SNPs. This process of rewashing was repeated 2 to 3 times to remove the unwanted substance over SNPs surface).
UV-Visible Analysis
The SNPs biosynthesis was determined using UV–Visible spectrometry. In 10 ml volumetric flask, 1 mg of dried SNPs was dissolved in 10 ml deionized water. Test mixture was analyzed by UV–Visible spectrometer at 200 to 800 nm to detect the surface plasmon resonance (SPR) peak.
Optimization of parameters for SNPs biosynthesis
The SNPs biosynthesis was optimized based on UV-Visible spectrometric studies over DLLAE and AgNO3 reaction mixture, maintained under different parametric conditions, such as: AgNO3 concentration, DLLAE and AgNO3 ratio, pH and temperature. The optimization of SNPs was conducted as per reported protocols with minor modifications (Fuloria et al., 2019).
All the reaction mixtures were visually examined (for color change) and subjected to UV-Visible spectrometry (to observe the SPR signal) after 24 h of incubation maintaining same reaction conditions except for individual parameters.
Optimization of AgNO3 concentration
To optimize the biosynthesis of SNP based on AgNO3 concentration, the SNPs biosynthesis involved preparation of five individual reaction mixtures with difference in AgNO3 concentration, such as: 1, 2, 3, 4, and 5 mM.
Optimization of volumetric ratio of DLLAE to AgNO3
To optimize the biosynthesis of SNP based on DLLAE and AgNO3 ratio, the SNPs biosynthesis involved preparation of nine individual reaction mixtures in nine different ratios of DLLAE and AgNO3, such as: 1:9, 2:8, 3:7, 4:6, 5:5, 6:4, 7:3, 8:2 and 9:1.
Optimization of temperature
To optimize the biosynthesis of SNP based on temperature, the SNPs biosynthesis involved preparation of three individual reaction mixtures with difference in temperatures, such as: 4°C, 25°C room temperature and 60°C.
Optimization of pH
To biosynthesized SNPs were optimized based on pH, the SNPs biosynthesis involved preparation of four individual reaction mixtures with difference in pH, such as pH 4, pH 5, pH 7 and pH 8. The reaction mixtures pH was adjusted using 0.1 N NaOH and 0.1 N HCl.
Stability study of biosynthesized SNPs
The stability of biosynthesized SNPs was determined on the basis of SPR signal range (340 to 540 nm). The readings were taken on 0 h, ½ h, 2 h, 24 h, 7 d and 15 d intervals.
SNPs characterization
The biosynthesized SNPs were characterized based on other standard research studies (Logeswari, Silambarasan, & Abraham, 2015). Before characterization, the synthesized SNPs were subjected to purification. This was done to avoid interaction of extract absorbance with nanoparticles absorbance. The characterization of SNPs was done using Fourier transformed infrared spectrometer (FT-IR), X-ray diffraction (XRD), Energy-dispersive X-ray (EDX) analysis instrument and field emission scanning electron microscope (FESEM). The formation of biosynthesized SNPs was determined based on a change in color of the solution, UV–Visible spectrometer (Shimadzu U-2800) and FT-IR (PerkinElmer SLE/MSC4/29) spectral data. The FESEM measurement was performed to understand the morphology of SNPs. The SNPs crystal nature was examined by X-Ray diffractometer. The EDX analysis was performed by FEI Nova Nano SEM 450 with EDX assembly.
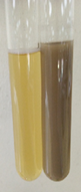
Antimicrobial activity of biosynthesized SNPs
The experimental method was based on other investigation studies with minor developments (Sharma, Yngard, & Lin, 2009). The biosynthetic SNPs were evaluated for antimicrobial potential against P. aeruginosa (ATCC 25619), B. subtilis (ATCC 6633), S. aureus (ATCC 29737) and E. coli (ATCC 8739) by well diffusion technique. The fresh and pure culture of each bacterial strain was sub cultured over nutrient broth (NB) at 37°C (previously shaken on a rotary shaker at 130 rpm). The culture of each bacteria was swabbed over nutrient agar plates. The wells of 8 mm size were drilled over nutrient agar plates. In each well of nutrient agar plate, using micropipette were added DLLAE (10, 20, 40, 60, 80 and 100 µg/mL), SNPs (10, 20, 40, 60, 80 and 100 µg/mL), and ciprofloxacin (50 µg/mL) each in with 50 µl volume. The inhibitory zones were measured after incubating the plates for 24 h at 37°C.
Results and Discussion
The SNPs biosynthesis was assessed visually and through UV-Visible analysis. The stirred mixture of AgNO3 solution and DLLAE was kept aside for 24 h at room temperature to monitor the color change. The solution color change to brown revealed the Ag+ reduction (Figure 1). The current study data established the successful biosynthesis of SNPs using DLLAE. Biosynthesis of SNPs occurred, when AgNO3 was exposed to DLLAE. Visual examination of color change confirmed the SNPs biosynthesis and Ag+ reduction. The color change was attributed to surface plasmon resonance property, conceivably a result of stimulation of longitudinal plasmon vibrations stimulation (Fuloria et al., 2019; Logeswari et al., 2015).
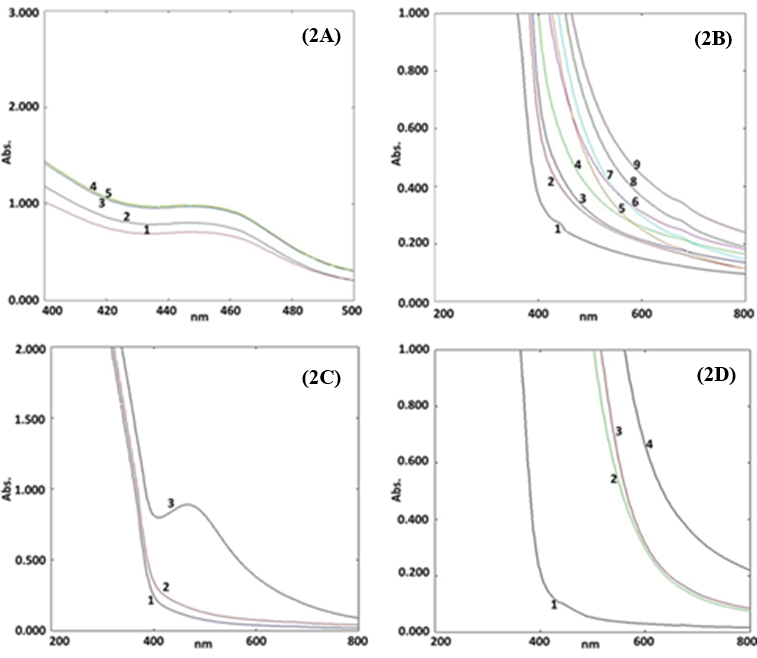
Optimization of parameters for SNPs biosynthesis reaction mixture
The optimization of parameters for SNPs biosynthesis reaction mixture was founded on: AgNO3 concentration, DLLAE and AgNO3 ratio, temperature and pH. In present investigation, the optimization data was validated based on SPR range of standard investigations (Erjaee, Rajaian, & Nazifi, 2017).
Optimization of AgNO3 concentration
The biosynthesized SNPs optimization based on five concentrations of AgNO3 (1, 2, 3, 4 and 5 mM) as parameter offered a UV-Vis. plot comprising five curves (Curve 1, 2, 3, 4 and 5) shown in Figure 2(A). The UV-visble spectrum exhibited the curves at 447 nm (1 mM), 445 nm (2 mM), 449 nm (3mM), 449 nm (4mM) and 450 nm (5mM). A shifting in SPR signal was observed on increasing the AgNO3 concentration.
The spectrum in Figure 2(A), revealed maximum absorption for 5 mM of silver nitrate solution (450 nm). Hence, 5 mM of AgNO3 concentration was considered as optimum for biosynthesis of SNPs. Optimization data for AgNO3 was also supported by SPR signal range of other research (Cecilia et al., 2016).
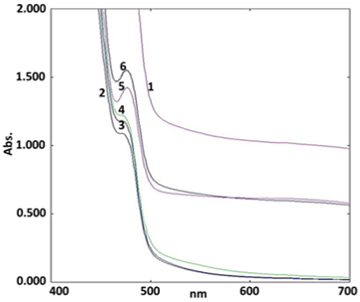
Optimization of volumetric ratios of DLLAE and AgNO3
The biosynthesized SNPs optimization based on nine ratios of DLLAE and AgNO3 (1:9, 2:8, 3:7, 4:6, 5:5, 6:4, 7:3, 8:2 and 9:1) as parameter offered a UV-Vis. plot comprising nine curves (Curve 1, 2, 3, 4, 5, 6, 7, 8 and 9) shown in Figure 2(B). Curve 1 represented the absorbance peak at 418 nm, which confirmed with the formation of SNPs. The other curves showed the absorbance peaks within the range of 650 nm to 656 nm, which were not fall within the range of SNPs formation. Therefore, the volumetic ratio of DLLAE to AgNO3 (1:9) was considered as optimum for biosynthesis of SNPs. The optimization study results for volumetric ratio of DLLAE and AgNO3 (1:9) were also supported by the SPR signal range of other research studies (Erjaee et al., 2017; Fuloria et al., 2019).
Optimization of temperature
The biosynthesized SNPs optimization based on temperature (4°C, 25°C and 60°C) as parameter offered a UV-Vis. plot comprising three curves (Curve 1, 2, and 3) shown in Figure 2(C). The UV-Visible spectrum, showed the effect of temperature on the synthesis of SNPs. The Figure 2(C) UV-Visible spectrum, exhibited no SNPs signals in curve 1 and 2 (4°C and 25°C). The spectrum exhibited SNPs signal in curve 3 (60°C) at 453 nm revealing the completion of SNPs synthesis. The absorption spectrum (Figure 2C) revealed that, room temperature and 4°C (representing curve 2 and 1) were not ideal to formulate SNPs. Whereas, 60°C (representing curve 3) was the ideal temperature to produce SNPs displaying signal at 453 nm. Hence, maintaining the reaction at 60°C was considered as optimum for SNPs biosynthesis. The results of optimization study over SNPs biosynthesis were also supported by other literary evidences (Erjaee et al., 2017; Shaik et al., 2018).
Optimization of SNPs synthesis reaction mixture for pH
The optimization of SNPs synthesis based on four pH (pH 4, pH 5, pH 7 and pH 8) yielded a spectrum in Figure 2(D), comprising four curves 1, 2, 3 and 4. UV-Visible spectrum, exhibited no signals for SNPs in curve 2, 3 and 4 (pH 8, pH 7 and pH 5). The spectrum displayed an SPR peak for SNPs in curve 1 (pH 4) at 416 nm revealing the SNPs synthesis. The Figure 2(D) UV-Visible spectrum produced on analysis of SNPs biosynthetic reaction at pH (4, 5, 7 and 8) revealed that pH 8, pH 7 and pH 5 (representing curve 2,3 and 4) were unsuitable to formulate SNPs. Whereas, pH 4 (representing curve 1) was ideal to produce SNPs displaying signal at 416 nm. Hence, maintaining the reaction at pH 4 was considered as optimum for SNPs biosynthesis. The pH optimization results for SNPs biosynthesis were supported by SPR signal range of other studies (Erjaee et al., 2017; Shaik et al., 2018).
Stability study for biosynthesized SNPs
The stability study of biosynthesized SNPs using UV-Visible spectrometry experiment was conducted for 0 h, ½ h, 2 h, 24 h, 7 d and 15 d respectively. The results of stability study after fixing time as revealed that SPR signal was retained in the expected range.
The Figure 3 illustrated of SNPs signal between 489 to 553 nm. It was observed that the spectra absorbance of SNPs was constant. Hence, stability studies showed that the SNPs were stable after 15 d. The present study SNPs signal range was also supported by other research studies (Erjaee et al., 2017; Shaik et al., 2018).
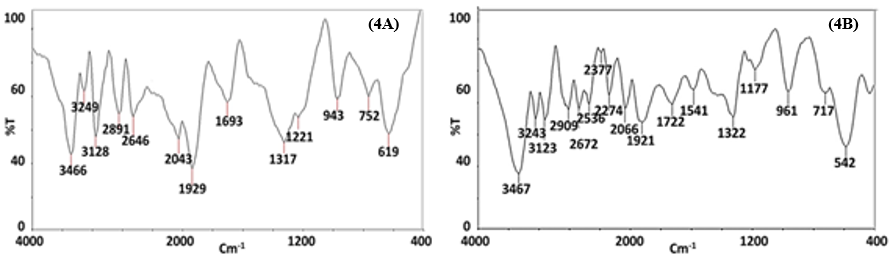
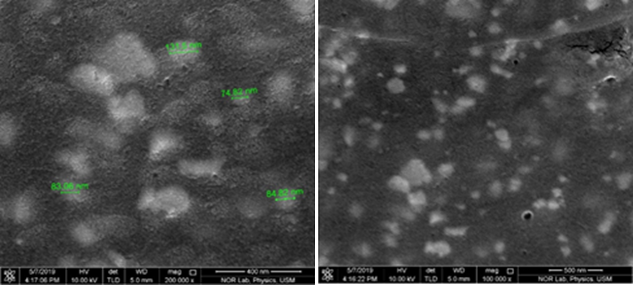
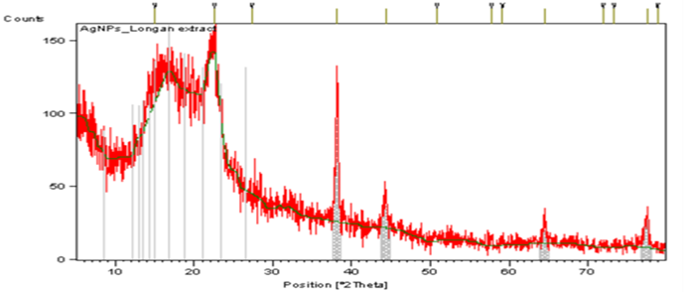
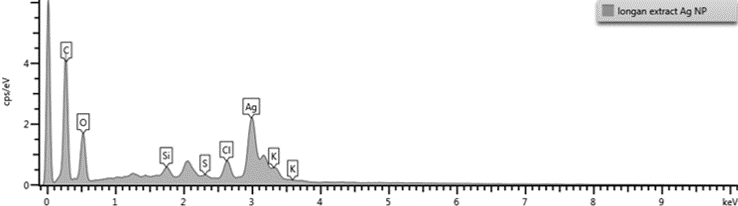
Microorganism |
Zone of Inhibition in mm |
||||||||||||
---|---|---|---|---|---|---|---|---|---|---|---|---|---|
SNPs |
D. longan Extract |
Ciprofloxacin |
|||||||||||
Concentration (μg/ml) |
10 |
20 |
40 |
60 |
80 |
100 |
10 |
20 |
40 |
60 |
80 |
100 |
50 |
B. subtilis |
18 |
18 |
18 |
24 |
23 |
25 |
N/A |
N/A |
N/A |
N/A |
N/A |
N/A |
21 |
S. aureus |
13 |
14 |
13 |
15 |
15 |
14 |
N/A |
N/A |
N/A |
N/A |
N/A |
N/A |
21 |
P. aeruginosa |
N/A |
N/A |
N/A |
20 |
22 |
23 |
N/A |
N/A |
N/A |
N/A |
N/A |
N/A |
21 |
E. coli |
14 |
14 |
14 |
15 |
15 |
16 |
N/A |
N/A |
N/A |
N/A |
N/A |
N/A |
20 |
N/A: No activity
Characterization of biosynthesized SNPs
Fourier Transformed Infrared (FTIR) analysis
FTIR spectroscopy is used to identify the characteristic functional groups of chemical moieties. The FTIR experiment over the DLLAE and SNPs offered the two spectra given in Figure 4(A) and Figure 4(B).
FTIR experiment determined the Ag+ to Ag0 reduction and SNPs synthesis. The DLLAE FTIR spectrum given in Figure 4(A) exhibited characteristic bands: 3466, 2891, 1693 cm-1, attributed to O-H, C-H and C=O cm-1vibrations respectively. IR spectrum of SNPs given in Figure 4(B), exhibited shifted and broadened IR bands at 3467, 2909, 1722, and 1541 cm-1 attributed to O-H, C-H, C=O, C=N vibrations.
The FTIR data revealed SNPs formation and Ag+ to Ag0 reduction of (Fuloria et al., 2019). Based on comparison of FTIR spectra of DLLAE and biosynthesized SNPs, the DLLAE was recognized as dual capping (stabilizing) and reducing agent. The Figure 4(B) IR spectrum of SNPs was similar to figure IR spectrum of DLLAE, as it preserved majority of signals with marginal shifting and broadening. For example, 3466 cm-1 (O-H vibrations) narrow band in Figure 4(A) FTIR spectrum of DLLAE was shifted to 3467 cm-1 as a broad band in Figure 4(B) FTIR spectrum of SNPs (Erjaee et al., 2017)
Field Emission Scanning Electron Microscopy (FESEM)
FESEM analysis is used to study the morphology of the biosynthetic SNPs (Mourdikoudis, Pallares, & Thanh, 2018). FESEM micrographs of SNPs are shown in Figure 5 with different magnifications. FESEM images showed that SNPs formed were polydispersed, spherical shaped. Particle sizes for SNPs ranged from 74.82 nm to 131.5 nm and were resulted by silver reduction by DLLAE from AgNO3.
X-Ray diffraction (XRD) analysis
The SNPs crystalline nature was analyzed by XRD pattern given in Figure 6. The diffraction peaks at 2θ values of 78.83, 64.47, 44.33, and 38.08 degrees can be indexed to face centered cubic structure of the silver reflection planes of SNPs. Apart from Bragg signals of silver nanocrystals, additional signals were also observed. These signals occur due to presence of biomolecules that are present in DLLAE. XRD results of SNPs were also verified by other studies (Ranjith, Arungandhi, Sunmathi, Priyanga, & Monisha, 2018). Average size of SNPs was determined to be 48.23 to 59. 06 nm using Debye–Scherrer formula (Yasir, Singh, Tripathi, Singh, & Shrivastava, 2017).
Energy dispersive X-Ray diffraction (EDX) analysis
EDX was used for elemental analysis to understand the presence of element involved in the SNPs development (Figure 7). EDX data revealed strong signal for silver and confirmed the SNPs formation. The silver (55.54%) was found as major element in comparison to carbon (21.16%), oxygen (14.72 %), and chlorine (3.84 %). Usually, SNPs exhibits signal at 3 KeV attributed to SPR. The EDX data exhibited strong signal for silver and weak signal for oxygen, that may be due attributed to biomolecules bounded to SNPs surface (Dakhil, 2017).
Antimicrobial activity of SNPs
The SNPs are extensively used being antimicrobial and registered as more potent in comparison to silver ions. As per standard protocol (Fuloria et al., 2019) the biosynthesized SNPs were investigated for their inhibition activity against S. aureus, E. coli, P. aeruginosa and B. subtilis. The zone of inhibition (ZOI) was observed for each plate and the diameter was measured in mm (data given in Table 1). The resultant data revealed that DLLAE possess no antimicrobial potential but when the same DLLAE was blended into SNPs, the SNPs of the same exhibited very strong antimicrobial potential.
The biosynthesized SNPs displayed highest zone of inhibition against B. subtilis (25 mm) in 100 µg/mL dose. The pure DLLAE displayed no zone of inhibition against B. subtilis, E. coli, P. aeruginosa and S. aureus (data given inTable 1). When compared to ciprofloxacin, the SNPs exhibited a highest inhibition zone against B. subtilis, P. aeruginosa, E. coli, and S. aureus that is 25, 23, 16, and 14 mm respectively at 100 µg/mL dose. Interestingly, a pattern was observed in the antimicrobial activity of newer SNPs, when the concentration of SNPs was increased from 10 µg/mL to 100 µg/mL there was substantial rise in zone of inhibition. The antimicrobial activity results indicated that capping of silver with biochemical moieties of DLLAE (sugars, proteins, steroids, anthraquinones and coumarins glycosides, flavonoids, alkaloids, organic acid, tannic and phenolic compounds) caused a marked increase in the antimicrobial potential of SNPs formulated from DLLAE. This pattern of increment in antimicrobial response due to biochemical moieties is also supported by other investigations (Fuloria et al., 2019). Hence with it can be hypothesized that small sized SNPs (biosynthesized from DLLAE), when increased in concentration from lower dose (10 µg/mL) to higher dose (100 µg/mL), leads to an increase in antimicrobial response. Also, in future, this method can be employed in drug delivery system attributed to its cost which is economical when compared with other conventional approaches (Pazos-Ortiz et al., 2017).
Conclusion
Current study was aimed to biosynthesize and determine the antimicrobial potential of SNPs of DLLAE. In this study, the SNPs were synthesized in eco-friendly way without using hazardous chemicals. The visual examination of color change to brown, UV-Vis and FTIR data confirmed the success of SNPs biosynthesis using DLLAE. The FESEM, EDX, and XRD data established the morphology of biosynthesized SNPs. The SNPs formed were poly dispersed and spherical in shape. The antimicrobial activity of SNPs formulated in present study establish that small sized SNPs (biosynthesized from DLLAE) when increased in concentration from low to high dose causes rise in antimicrobial response. Hence, present study concludes that silver nanoparticles obtained from DLLAE strong response against B. subtilis, E. coli, S. aureus, and P. aeruginosa. Study recommends DLLAE as potential resource to produce the potent antimicrobial SNPs.