A Comprehensive Review on Current Progressions in Combating The Antimicrobial Resistance
Abstract
Anti-microbial resistance (AMR) is the ability of a microbe to resist the effect of antibiotics. It has become the most important cause of concern throughout the world. AMR may develop a variety of mechanisms, including resistance, weakness, or due to a certain therapeutic failure. The three major causes of resistance were identified to be over usage of anti-microbials, over-prescription, increased agricultural usage. The mechanism which leads to the development of AMR was found to be Metabolic Inactive Persister cells biofilm formation, Swarming, Obligate intracellular microbes, Reduced uptake and efflux pumps, Modification of the antibiotic binding site, Production of competitive inhibitors. Research is still being carried out and few methods successfully delay or inhibit the progress of Resistance namely Photodynamic therapy, which works on the principle of photosensitization, results in formation of ROS which destroys pathogenic cells, Vaccines, which strengthen the defence system to fight against foreign microorganisms efficiently, Antibiotic adjuvants, work by inhibiting any kind of mechanism related to the resistance increasing the drug’s antibacterial efficiency, Nano antibiotics which acts by interfering with the mechanisms for the development of the resistance and microfluidics give a better understanding of the bacteria and its superior antimicrobial susceptibility testing. Hence, in this review, the above mechanisms of antimicrobial resistance as well, the latest AMR combating strategies are discussed.
Keywords
Antimicrobial resistance, Therapeutic failure, Photodynamic therapy, Vaccines, Nano antibiotics, Microfluidics
Introduction
AMR has emerged as one of the leading phenomena of the 21st century, majorly regarding bacteria. The mechanism of this resistance may be acquired or mutated depending on the resistance or vulnerability. Another term related to this resistance known a clinical resistance, which is caused mainly due to the therapeutic failure of the drug in a higher probability scale. In order to understand the clinical resistance, it is important first to analyze the clinical setting, the specific position of infection, or the dose at which the drug was taken. It is known that microorganisms have managed to survive for over a million years, mainly due to the property of Adaptivity in any kind of hostile environment.Antimicrobial resistance in the pathogenic bacteria has led to a worldwide challenge related to high morbidity and mortality. The both gram-negative and gram-positive bacteria have seen the multidrug-resistant patterns and led to untreatable infections with conventional antibiotics. It has become a bigger challenge for the health sciences community to find a way to act against the resistance. Many of the infections caused by the resistant microorganisms do not respond to the traditional antibiotic treatment. The development to combat the resistance is vastly under pressure and the new medications that enter the market are expected to be used a small amount to prevent the development of the resistance and the diagnostic tools will guide the treatment. There is a need to develop a novel and alternative solution (Hengel & Marin, 2019).
Cause for Antimicrobial Resistance
Two causes, namely human and the intrinsic characteristics of the microorganism, are depicted in Table 1 and Table 2 given below.
The current methods against the antimicrobial resistance include the following
Photodynamic Therapy
The photodynamic therapy (PDT), works on the principle of photosensitization. In the presence of oxygen, there is the formation of reactive oxygen species which destroys target pathogens PDT has been used as an efficient tool for treating infectious and cancer conditions (Li et al., 2019).
Mechanism
Two mechanisms have been in the photodynamic action Type I and type II (see Figure 1). At the ground state, the photosensitizer absorbs the photon and gets transformed into its triplet state via a single state. The triplet state so formed is the reactive intermediate. In the first mechanism, the triplet state photosensitizer transfers its electron to the ground state to form a reactive oxygen species (ROS). These ROS can be in the form of superoxide, hydroxyl radicals and peroxides. In the second mechanism, the triplet state forms another ROS, which is very reactive singlet oxygen. The formed reactive species react with the nearby biomolecules like bacterial cells to give the bactericidal action (Li et al., 2019).
Due to human |
Reference |
---|---|
Overuse of antimicrobials 1. The remarkable effectiveness of antibiotics has led to their widespread usage. 2. The universal usage of the antibiotics has caused the increased application and applied strong, widespread and selective pressure on the world of microorganisms which certainly has caused an increase in the rate of resistance observed today. |
|
Over prescription 1. The continuous prescription of antibiotics has led to the widespread of the AMR. 2. This casual use of antibiotics is more or less due to inadequacies in quick and accurate analysis of the pathogenic diseases |
|
Increased agricultural use The antibiotics used in livestock are ingested by humans when they consume food.
|
Mechanism due to characteristic of the bacteria |
Example |
References |
---|---|---|
Metabolic inactive persister cells biofilm formation: There is a tolerance to antibiotics due to the presence of persister cells in bacteria. Hence when bacteria are exposed to antimicrobials the cells which are sensitive to the drug will be removed and presister cell will be unaffected causing infection to reappear Biofilm is a protective film composed of proteins and these films are less sensitive to antimicrobial treatment.
|
S. aureus and P. aeruginosa |
|
Swarming This leads to tolerances in the organism. Planktonic cells Swarm cells (antibiotic tolerant) |
Bacillus subtilis, Serratia marcescens, E. Coli, Salmonella typhimurium |
|
Obligate intracellular microbes These obligate microbes are kept safe from the antimicrobials due to their limited capability of the chemotherapeutic agents the host cell. |
Mycobacterium leprae, Chlamydia, non-Bartonella Rickettsiae |
|
Reduced uptake and efflux pumps This mechanism helps in preventing the concentration of the antibiotic from increasing in the bacterial cells. |
P.aeruginosa and E.coli |
|
Modification of antibiotic binding site The sites of the substrate to which antibiotic binds get modified due to expression of certain drugs which causes a decrease in the antimicrobial activity |
MecA gene in beta lactums |
|
Production of competitive inhibitor In some bacteria, there will be a production of certain compounds which will inhibit the drugs action by preventing it from binding of the drug to its target site |
PABA formed in S. aureus and N. meningitidis inhibits sulphonamide drugs to bind to its target site, i.e., dihydropteroate synthetase |
Photosensitizers |
Organism |
Disease condition |
Reference |
---|---|---|---|
Phenothiazinium |
Fusarium keratoplasticum and Fusarium moniliforme |
Mycosis |
|
Photoditazine Fotoenticine |
Streptococcus mutans |
Dental caries |
|
Methylene blue Indocyanine green |
Candida albicans, Herpes simplex virus |
Genital herpes |
|
Aminolevulinic acid |
Human papillomavirus |
Epidermodysplasia verruciformis |
|
Endogenous porphyrins |
Corynebacterium minutissimum |
Erythrasma |
|
Toluidine blue o |
Helicobacter mustelac |
Stomach ulcer |
|
Aminolevulinic acid |
H.pylori |
Stomach ulcer |
Type of the |
Name of the nanomaterial |
Bactericidal action |
Target organism |
---|---|---|---|
Goal based nanomaterial |
AuNRs |
PTT |
E. coli E. faecalis S. aureus S. mutans S. oralis |
AuNRs Cluster |
PTT |
E. coli |
|
AuNSs |
PTT |
MRSA |
|
Carbon based nanomaterial |
FGO-FA + AgNPs |
PTT & silver ion release |
E. coli S. aureus |
GO-IONP-Ag |
PTT & silver ion release |
S. aureus |
|
Fe3O4@GO-QCS |
Bacteria Capture, PTT & Magnetic Recycle |
E. coli S. aureus |
|
Metal based nanomaterial |
CuS-PVP |
PTT |
S. aureus |
BSA-CuS |
PTT |
S. aureus E. coli |
|
Fe5C2 |
PTT |
S. aureus E. coli |
Vaccine |
Company |
Species of Organism |
Composition |
Status Of Clinical Trial |
---|---|---|---|---|
PF-06425090 |
Pfizer |
C. difficile |
Genetically/ chemically deactivated C. difficile toxins A and B |
Phase 3 |
ACAM-CDIFF |
Sanofi |
Formalin-inactivated wild-type toxoid (A and B) ACAM-CDIFF |
Phase 3 |
|
VLA84 |
Valneva |
Recombinant fusion protein containing of truncated toxin A and B |
Phase 2 |
|
SA4Ag |
Pfizer |
S. aureus |
CP5/CP8-CRM197, P-Y variant ClfA, MntC |
Phase 2b |
4C-Staph |
GSK |
Csa1A (Sur2), FhuD2, EsxA/EsxB, HlAH35L |
Phase 1 |
|
EcoXyn-4V |
GlycoVaxyn |
E. coli |
E. coli bioconjugate vaccine |
Phase 1 |
FimH adhesin vax |
Sequoia |
Protein-based vaccine |
Phase 1 |
|
JNJ63871860 |
Janssen |
E. coli bioconjugate vaccine |
Phase 2 |
|
Trivalent GBS vaccine |
GSK |
Group B Streptococcus |
Capsular epitopes of GBS serotypes Ia, Ib and III conjugated to CRM197 |
Phase 2 |
Bivalent GBS protein vaccine |
Minervax |
N-terminal domains of the Rib and alpha C surface proteins |
Phase 1 |
Type Of Nanomaterial |
Example |
Target Organism |
Reference |
---|---|---|---|
Carbonnanotube (CNT) |
Functionalized CNT |
Leishmania |
|
Fullerenes |
Sulfobutyl fullerene |
Environmental bacteria |
|
Dendrimers |
Caumarin derived dendrimer |
Plasmodium falciparum |
|
Nanocomposites |
Silver bio nanocomposite |
Gram-positive and gram-negative bacteria |
|
Chitosan silver oxide nanocomposite |
E.coli and staphylococcus aureus |
The increasing number of chronic UTIs which is caused by E.coli, a gram-negative bacterium, and over-application of chemotherapeutic agents has caused resistance in the species of E.coli. This had further led to the discovery and improvement of alternative ways for the inactivation of the microorganisms. Antimicrobial photodynamic therapy has been used in these conditions for the eradication of the both gram-negative and gram-positive microorganisms. Unlike antibiotics, which target a single major target, PDT targets a variety of bacterial cell structures and components. Hence PDT can reduce the risk of developing resistance of organisms exposed to it (Tichaczek-Goska, Wojnicz, Symonowicz, Ziółkowski, & Hendrich, 2019).
Photodynamic antimicrobial chemotherapy (PACT) will be effective in treating infections without antibiotics. For photodynamic therapy, no specific ligand-receptor interactions at or inside the bacteria is necessary and also no specific extracellular or intracellular localization of the photosensitizers is needed. The oxidative burst, which is induced during therapy, has no specific target site (Maisch, 2015). Compared to other conventional therapy like antibiotic treatment, PDT has several advantages. The ROS produced can kill the organism either by membrane damage, which leads to cellular content leakage or enzyme disruption or by cleavage of DNA. The other benefit of this therapy is that it has reduced the side effects due to the restriction of the treatment to the specific region being exposed. Also, this therapy has been used along with other antibacterial therapy like photothermal therapy, radiotherapy and other conventional treatment using antibiotics to improve the efficiency of the therapy. There are some challenges during the PDT therapy like firstly ROS that are generated have short lifetimes and the diffusion distances are restricted (<40 ns and 10nm respectively). Sometimes, ROS, which is produced by the photosensitizers, have very high non-specificity, which decreases the efficiency of the antimicrobial action. Secondly, the light which is being used to trigger the photosensitizer is not suitable for penetration deeper into the tissues (Li et al., 2018) .
Some of the infectious conditions which are treated effectively by the photodynamic therapy and the photosensitizers used are depicted in Table 3.
Photothermal Therapy
This is a light promoted thermal therapy for the bactericidal action that happens as a significance of quick localized heat produced by the Photothermal agent. This photothermal therapy (PTT) therapy is applied in the microbial infection and tumour therapies. Temperature based therapies are not known to cause antibiotic resistance and the drug-resistant bacteria are of severe threat, so PTT has been widely used recently (Yang, Cai, Huang, Tang, & Zhang, 2018).
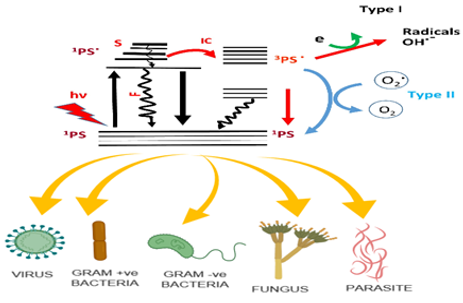
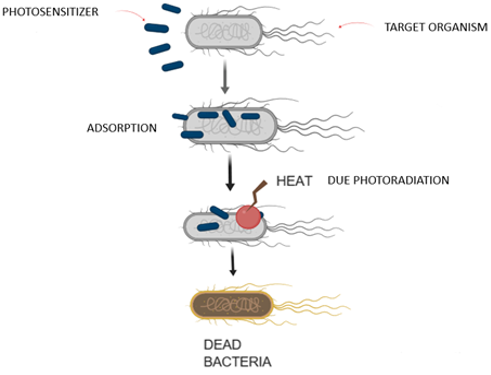
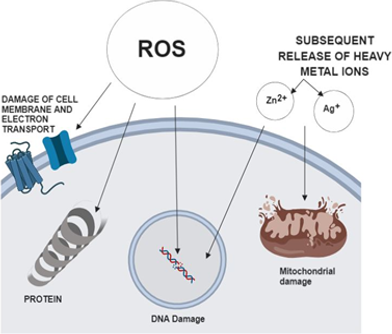
Mechanism
There are a series of steps in the PTT. (see Figure 2)Adsorption of the photothermal molecules on the bacterial surface by interactions such as hydrophobic, electrostatic, and hydrogen bonding. The interactions are so strong that it prevents nonspecific effect on the other normal cells.Due to photo radiation, the agent converts light into heat, which leads to an increase in the temperature of the exposed bacterial surface. The surface enzymes of the bacteria get denatured as the temperature is increased to 50°C that causes the ordinary cellular reactions to stop leading to the death of the bacteria. The denaturation is a nonspecific activity and hence it is hard for the bacteria to give the remedial response and the development of resistance is decreased (Pan et al., 2016).
The main advantage of PTT is that there is no resistant to the drug on the organism and the photo radiation applied have the longer wavelengths and can pass through deeper tissues. Also, the adverse effects on the human tissues can be reduced due to the restriction of the particular region to the light irradiation. The main challenge for the photothermal therapy is that the direct heating of the bacterium is hard and hence to recognize the specific bacterium would improve the efficiency of the treatment. Also, if the interactions between the photothermal agents and the micro-organism are not that strong enough, the agent might get delocalized from the target micro-organism and cause damage to the other normal cells. Recently the supramolecular systems are developed to counter these shortcomings (Li et al., 2018).
Some of the nanomaterials which are known to have used in the photothermal therapy are illustrated below (see Table 4) (Teixeira et al., 2018).
Enhancing the Role of Vaccines
The present analysis of AMR has dedicated attention to its adverse effects on the health and would likely to grow in the near future (Andrews et al., 2019).
This analysis has resulted in the action plans to address this problem. These action plans include minimizing the antimicrobial use and development of new antibiotics. Nowadays, vaccines are used as a part of a remedy for the AMR. Vaccines perform by making the defence system such that it could identify and act against the foreign microorganisms in a swift and efficient way and thereby avoiding the development of the infectious disease or else by reducing the severity of the disease. The protection of the unvaccinated people who cannot be vaccinated can be protected by herd immunity by avoiding the disease and transmission of the pathogen is broken up which adds advantage when the vaccines are largely used in the population (Lipsitch & Siber, 2016). Some of the vaccines used against the microorganisms and its composition are given the Table 5 (Jansen, Knirsch, & Anderson, 2018). Types of vaccines efficient in preventing AMR includes
Type b Haemophilus influenza vaccine
These vaccines were the first beneficial vaccines that showed effective performance in preventing invasive disease in the immunized infants, older children and significantly decreased antibiotic use and thereby inhibiting the anti-microbial resistance.
Hib had effectively developed protection from ampicillin, driving proposals to utilize chloramphenicol and wide range cephalosporins for the exact treatment of meningitis. It thereby decreases the requirement for anti-microbials and avoids the development of multidrug resistance (Jansen et al., 2018).
Pneumococcal vaccines
Vaccinations have reduced the drug-resistant disease caused by the pneumococcus infections by targeting the most resistant serotypes. PVC7, a multivalent pneumococcal vaccine introduced in the US, reduced the penicillin non-susceptible c and also reducing the rate of multidrug-resistant invasive pneumococcal disease by 84%. Many other countries have also seen a decline in resistant invasive pneumococcal disease (IPD) by this vaccine introduction. Reduced antibiotic use directly reduces the AMR (Reinert, 2009).
Staphylococcus aureus vaccines
S. aureus has been the major cause for the infectious conditions like pneumonia, endocarditis, osteomyelitis and bacteraemia.S.aureus has developed resistance to the recently introduced antibiotics like linezolid that halts the production of protein in the bacteria (gram-positive) and disruption of the membrane due to daptomycin (Dayan et al., 2016).
The drug of choice for the infections due to the multidrug resistance S.Aureus, vancomycin has shown a reduction in the susceptibility (Jansen et al., 2018). S.aureus, which is known to cause resistant to a various classes of antibiotics, there is a strong desire to develop active vaccines for the protection against the strains of S.aureus and to reduce the occurrence of the resistance. Understanding the mechanisms of the S.aureus has resulted in the development of the new vaccines, which are present in the development (Giau, An, & Hulme, 2019).
SA4Ag a vaccine against the S. aureus infections has been effective in the fight against those organisms. This is an advanced vaccine that is being assessed in a phase 2 and 3 efficacy study for the prevention of invasive diseases caused by the S.aureus. This vaccine is known to be harmless and tolerated well during the early phase of clinical trials and also produces antibacterial antibodies is increased level in adults (Frenck et al., 2017).
Antibiotic Adjuvants
Another name for such compounds are ‘resistance breakers’ or antibiotic potentiators due to the fact that they possess faint or no apparent antibiotic activity whereas when administered along with other antibiotics, they possess two mechanisms of action (González-Bello, 2017).
1. Inhibit any kind of mechanism related to the resistance.
2. Increasing the drug’s antibacterial efficiency.
This combined drug therapy is a much cheaper alternative in drug discovery and may help in identifying relatively new targets that are essential in bacterial survival.
The main challenge in translational medicine is the increase in multidrug-resistant pathogenic bacteria, which includes Staphylococcus aureus, Enterococcus spp. Klebsiella spp., Pseudomonas aeruginosa, Enterobacter spp and Acinetobacter baumannii, which eventually makes it difficult to design newer drugs due to the rise in mortality and morbidity in developed countries (Boucher et al., 2009).Lately, several strains of gram-negative bacteria have also been found to show enhanced resistance to available antibiotics (Duin, Kaye, Neuner, & Bonomo, 2013).
Due to the increase in bacterial resistance, research is still being carried out in formulating novel adjuvants for combating it. Further compounds which are to be discussed further are inhibitors of the β-lactamase enzyme, inhibitors of efflux pump, and outer membrane permeabilizers (Gill, Franco, & Hancock, 2015).
Inhibitors of β-lactamase enzyme
Mechanism of action of this class of drugs is by inhibiting the proteins which lack specific mammalian homologs, namely penicillin-binding proteins. Based on the success of clavulanic acid, it was identified that the combinational therapy of β- lactamase and β- lactam antibiotics had shown an increase in the efficacy and spectrum of anti-microbials and immense research has also been carried out for the development of newer non-antibiotic β-lactamase inhibitor in combination with β-lactams. An example of such a combinational treatment is the co-administration of Penicillin along with these inhibitors such as, sulbactam, clavulanic acid, or tazobactam (Bush, 2012).
Certain newer drugs have been developed for the same purpose, namely Avibactam produced by AstraZeneca, which was first produced by Novexel. This drug possessing the structure bicyclic diazabicyclooctane, which is a non-β -lactam in nature, although it does not show any significant bactericidal activity, forms covalent acylation with β- lactamases. Since tazobactam and clavulanic acid is been known to have poor action against the class A and C strains of β-lactamases, Avibactam have been taken into consideration because it can do the same with great efficiency against the extended-spectrum b-lactamase Klebsiella pneumonia carbapenemase which is the common β-lactamase producing organisms (Bush, 2015).
In addition, some of these antibiotics must show action synergistically with β- lactams in invitro as well as in vivo studies. Caution must be taken to avoid toxicity by carefully titrating both the doses of β- lactam and β- lactamase inhibitors and hence clinical trials pose hurdles for such drugs.
Agents that inhibit efflux pumps
A mechanism of active transfer of certain drugs like tetracycline, which is a usual ingredient in the mechanism of antibacterial resistance, was first studied by McMurry et al. and it was identified as a wide phenomenon in the case of antibiotics which decrease the efficiency 1-64 fold. Due to the rapid increase in multidrug resistance (MDR), the discovery of antibacterial and resistance modifying agents are under study, by understanding the various mechanisms of resistance, including target site modification, antibiotic inactivation and decrease in cytoplasmic antibiotic concentration. It was also identified that bacteria can decrease the accumulation of antibiotics by two mechanisms, namely decreasing the permeability or increasing the efflux of antibiotics (Kabra, Chauhan, Kumar, Ingale, & Singh, 2019). In the case of Gram-negative bacteria, Resistance-Nodulation- Division (RND) efflux pumps are involved in intrinsic resistance which, when activated, led to resistance to the multidrug resistance in the species like Pseudomonas aeruginosa and Enterobacteriaceae hence these are used as a novel goal which could restore susceptibility to various antibiotics. Another hypothesis suggested for the reduction of harmful outcomes of these pumps is the use of antisense peptide nucleic acids (PNAs), which are nucleic acid homologs (synthetic) where a replacement of polynucleotide phosphate sequence is observed with flexible pseudopeptide polymer. Its mechanism involves binding with complementary DNA and RNA with high specificity, thereby inhibiting gene expression followed by a translation (González-Bello, 2017).
Outer membrane permeabilizers
The complex structure of the Gram-Negative bacterial membrane, consisting of the extra layer made up of polyanionic lipopolysaccharides, is one of the main reasons for the limited efficacy of antibiotics. In such cases, permeabilizers may be used to improve antibiotic uptake, by cationic or amphiphilic chelators, which decrease the stability of the external layer of the membrane by interaction with polyanionic lipopolysaccharides or capturing of the positive presence in the outer layer hence facilitating the uptake of the drug. Examples of outer membrane permeabilizers are polymyxins, such as colistin, aminoglycosides, cationic peptides, cationic cholic acid derivatives, or polyamines. The external layer is a semipermeable wall including asymmetric two layers consisting of porins which are network that produces proteins. The area of the channel through which hydrophilic drugs such as βlactams, which can pass through is relatively small (<1% of the surface area) and hence restriction in uptake into the cell, which causes other mechanisms for the resistance. The presence of uncommon polyanionic molecule lipopolysaccharide (LPS) which is made stable by cross bridging of divalent cations, has also been observed in the outer membrane which serves as a barrier to restrict the movement of the drugs which are hydrophobic Several agents such as polycations namely aminoglycoside, polymyxins, cationic antimicrobial peptides and dibasic macrolides have the ability to destabilize the outer membrane and hence making it susceptible to the permeability of hydrophobic and hydrophilic substances which is termed as 'self-promoted uptake.' Hence this process enhances the permeability of antibiotics (Gill et al., 2015).
Though several researches have been carried out, the complexity of prokaryotic and eukaryotic membranes due to the presence of different lipids, creates problems with the use of certain permeabilizers (particularly cholic acid and its derivatives) due to absence of bacterial specificity whereas, in case of polymyxin B nonapeptide, signs of toxicity have been identified in early clinical trials. Certain compounds also cause alterations in the lipid metabolism and hence made unsuitable for human use (Melander & Melander, 2017).
Nano antibiotics against AMR
Nano antibiotics may be defined as nanomaterials that show anti-microbial potential or show synergistic potential with antibiotics administered (Zhang et al., 2018). These antibiotics have been tested invitro as well as in vivo methods. These materials do not show direct and short-term adverse effects though long-term exposure effects are not that well known. It has a significant advantage as they are cost-effective and tackle multiple pathways and it is not easy for the organism to develop resistance against NP microbial activities. It is stable enough to withstand harsh conditions and maintain prolonged shelf life, whereas conventional antibiotics would generally have been inactivated.
A number of hypotheses regarding the mechanism of metal and metal oxide NPs include (Zhang et al., 2018).
-
Physical disruption of cell structure
-
Generation of Reactive Oxygen Species (ROS) and subsequent depletion of anti-oxidants (see Figure 3).
-
Dysfunction of proteins
-
Disruption of transduction pathways by dephosphorylation of peptide substrates
-
Suppression of bacterial growth
Advantages
It is a promising treatment in the design of effective therapeutic agents against several strains of bacteria, including pathogenic varieties and useful in overcoming resistance. Nanocarriers may be engineered to perform target-specific delivery of antibiotics NPs may be modified to optimize the physicochemical parameters and subside maximum possible side effects during systemic administration.NP based drug delivery shows improved solubility and suspension of drugs and subsequent delivery of a number of agents in antimicrobial therapy with similar mechanisms (Sikwal et al., 2016).
Disadvantages
Evaluation of interactions of nano antibiotics with cells and tissues require several changes in the dosage in order to obtain the desired therapeutic effect. Extensive data about the toxicity data are also required to acquire success at the clinical level. Certain toxicity levels have been observed due to accumulation in colon, lung, bone marrow, etc. Inhalation NPs enter into the systemic circulation and may affect the lungs, liver, spleen, brain due to the ability to cross the epithelial and endothelial cells (Zhang et al., 2018).
Future prospective
Metal nanoparticles due to its ideal properties have shown to be of interest in constructing nanomaterials in antimicrobial therapy. These nano antibiotics may be an effective substituent for traditional antibiotics. Though no FDA approvals have been obtained for the same for human use, future studies are still being carried out to determine the physicochemical and pharmacological aspects to understand the safety of such products.
The latest research is being carried out in the combinational study of metal NPs and antibiotics in targeting the drug-resistant bacterial planktons and may be used in the near future to focus on antimicrobial resistance (see Table 6). Conclusively, Nanoparticles may serve as therapeutic agents in the near future in targeting superbugs and efforts are still being put in to commercialize nano antibiotics to improve the quality of life.
Microfluidics as A Tool to Fight Against AMR
Due to the restrictions of standard AMR techniques mentioned on top of, microfluidic-based platforms are developed to reinforce understandings of microorganism resistance, give sensible AST and MIC testing tools, and development of the new medicine. This review aims to summarise the recent developments in microfluidic-based AST and MIC testing tools in several formats of microfluidics technology like the detection of organic chemistry and physiological changes in microorganism culture. Antimicrobial status testing (AST) could be a crucial technology to realize data relating to microorganism ohmic resistance towards medicine and, ultimately, control the unfold of AMR. Many microfluidic-based platforms have established that AST will give quicker results, whereas guiding clinical selections of antibiotic use and up patients’ health, for example, in an every microfluidic-based AST and MIC study, morphological changes of single microorganism cells were tracked. Moreover, 189 clinical isolates were studied, and MIC was achieved among four hours that was a big reduction in operative time. In another study allotted with a similar platform, associate AST of Mycobacterium tuberculosis was achieved in nine days that's nearly six-fold reduction in microorganism growth in standard technologies (Hassan & Zhang, 2018). Microfluidics will help in the prevention of AMR in the following ways
To get to know more about bacteria
The general mechanism of the resistant of the organism to the antibiotic are known, but information regarding the other characteristics of the bacteria is still incomplete and the additional knowledge of those can be of immense importance in the effective understanding of AMR hence resulting in more suitable solutions. The usual methods which use conventional plates and tubes do not answer the most complex doubts of microbiology and the microfluidic technology has been known to have high versatility as well as efficient in this regard. For example, the reason behind the heterogenous development of the mycobacterium was understood that it is an unusual elongation of certain cells using this system, which can track the microbes at the cellular level. The microfluidics have been used to understand the dynamics of the persister cells, which are often responsible for AMR in the therapy of tuberculosis. This information regarding the persister cells can help in the development of the new strategies for the treatment of such diseases. Microfluidic systems have also been effectively used in the screening of the individual bacterial cell for the formation of some precise chemicals and the biomechanical characteristics which might be associated with the AMR and cannot be possible using the current methods (Liu, Banaei, & Ren, 2017).
Superior Antimicrobial Susceptibility-Testing
The susceptibility testing has the key role in the AMR progression, since it quantifies the amount of resistant in the selected strain. Typical AST is widely used in the but lacks behind in the accurate, fast and efficient testing. To deal with these problems, various recent research studies have demonstrated using the microfluidics to develop newer AST tools. The quick susceptibility testing using microfluidics helps in timely antibacterial treatment and thereby enhancing the patient’s response to the antibiotic. Some of those devices are verified to show better outcomes, but yet there is substantial space for improvement. Certain innovative techniques used by the microfluidic device have helped it for certain morphological changes at the cellular levels. These devices are effective in determination of the MIC as well as the information regarding the rate and dynamics of the process of inhibition. Validations of dynamic models of antimicrobials and assessments of their outcome on bacterial cells can likewise be shown in this way, gaining the valuable data with rapid resolution. Microfluidics have been also used in the development of the newer antimicrobials. Emerging the novel antibiotics has been an important way for the battle against antimicrobial resistance; nonetheless, it is hard to develop one, since the synthetic methods did not lead to the expectations. The advanced property of microfluidics is often its major softness and also there is a need to identify that significant difficulties remain for microfluidics, in fighting AMR. The efforts and sufficient time are needed to avoid the technical issues of the innovation. Also, there are no much of the commercial outcomes because of its complex nature (Liu et al., 2017).
The application of the microfluidics is developing but needs immediate largescale conversion of it into commercial products. With no exemption, the antimicrobial resistance has an immense role for the microfluidics.
Conclusions
The increasing resistance of the microorganisms to the antibiotics has triggered a massive crisis in the treatment of infectious diseases. The therapies such as PDT and PTT, which does not use antibiotics have shown good efficacy in treating the infectious diseases. However, since the delivery of the light to the body is known to be a localized process, the treatment with PDT was found to be more likely to be applied, especially for localized diseases and not for systemic infections. The treatment with PDT has been slow but steady. The use of PDT in the effective treatment of periodontitis has given a ray of hope to treat various other infectious conditions. The use of adjuvants have enabled us to use the existing drugs on the organisms that have developed the resistance for a very long time or never had resistance in the first place. It utilizes the adjuvant and antimicrobial concurrently. The chance of developing resistance has been decreased. Vaccines and antibiotics have been widely used in modern medicine. The use of vaccines prevents the occurrence of infections and thus minimizes the use of antibiotics and thereby reduces the AMR. As evolution begins to erode the value of antibiotics, a multipronged approach to preserving and restoring this value is needed. Vaccines have an important role to play in doing so. Nano antibiotics are stable enough compared to the conventional one and metal nano antibiotics have shown marked action against the resistant strains. Microfluidics have been used for understanding the mechanisms of the resistance of various organisms to the antimicrobials, which in turn helps to understand the ways to control it. While the novelty of microfluidics makes it stand out, practical and commercialized products that offer significant advantages in dealing with critical issues are more important and needed. Actively targeting such market niches will help microfluidics getting past the obstacles to better practical employment, rather than staying on proof-of-concept research papers. As the examples in this article have shown, microfluidics has shown great potential and promise in combating AMR. The next step should be to make those research output into real products that can reach end-users and integrate into the existing settings of health-care and pharmaceutical research and development. Thus, employing alternative treatment methods such as those discussed above for bacterial diseases would help to decrease the amount of antibiotics being used and may lengthen the time required for antimicrobial resistance to develop in some species.