Effect of multiple emulsion on mortality of drosophila virils
Abstract
The frequent use of insecticides has led to the development of insecticide resistance by many insects. In some insect populations, resistance can be developed to multiple pesticides. Up to this, insecticides resistance became a key challenge, and the amounts of the used pesticides must be as lower as possible. In this study, we measured the potency of multiple emulsions on the mortality of Drosophila virils and the ability of the fly to ingest and excrete the emulsions as a new strategy to avoid the great use of pesticides. The obtained data showed that the value of the lethal concentration of half the number of individuals decreases when the exposure duration increases, which demonstrates the sensitivity of the larvae to the toxic effect of the formulation. This can help to suggest that this type of material can be more effective and lasting effect for as long as possible. Moreover, the ingestion and excretion of the formulated product in the hind-gut can help to suggest the ability of the studied formulation to pass through the peritrophic membrane and the midgut acidic regions as two important barriers. In conclusion, the formulated microcapsule has a satisfactory insecticidal potency against D. virilis in a dose-dependent manner which might help to avoid the developed resistance to commonly used insecticides.
Keywords
Drosophila virils, Insecticides, Multiple emulsion, Mortality, Peritrophic membrane
Introduction
In agricultural practices, farmers are always trying to protect their crops from the attacks of insects, weeds, nematodes, and pests. However, due to constant application, these pesticides enter the food chain through various ways, such as water, air, and soil and severely affect human health because most of which are carcinogenic and cytotoxic (Saini, Bagri, & Bajpai, 2017). As environmental contamination, bio accumulation, and increases in pest resistance are among the key challenges in the use of pesticides, so the reduction of the amounts of used pesticides as much as possible is critically needed (Balaure, Gudovan, & Gudovan, 2017). Nanotechnology, as a recent attractive tool was remarkably used in this regards through the formulation and delivery of active pesticide ingredients, as well as novel active ingredients, collectively referred to as nano pesticides. Moreover, improvement of the water solubility of poorly soluble pesticide active ingredients to improve bioavailability, which involves the formulation of the active ingredients into nanoemulsions are a promising tool in the field of pesticide delivery.
Fly fruit Drosophila has been repeatedly used as an in vivo model system to study pesticides toxicity. Pesticide administration to the fly usually involves feeding in an agar-gelled feed fly's medium (AM). Based on the fact that this method has several problems such as uncertainty or incorrect bioavailability of the amount of ingested pesticides, there is great interest in developing new methods of exposure the flies to pesticides. A recent method had been developed by (Soares et al., 2017) in which they successfully deliver food to the flies Continuous liquid feeding method at much higher concentrations than the AM method.
The Drosophila gut is divided into three different regions known as the foregut, midgut, and hindgut. Ingested foods are stored in a part of the foregut known as the crop which is present only in adult fly and then pushed into the midgut by the pumping force. It is well-known that the midgut is the main part of the gut for digestion and absorption of the ingested foods (Buchon et al., 2013).
It is interesting to know that some strains of Drosophila became less sensitive to most of the common insecticides such as neonicotinoids nitenpyram, imidacloprid, and thiamethoxam (Perry, Heckel, Mckenzie, & Batterham, 2008; Perry, Mckenzie, & Batterham, 2007). In addition, other D. melanogaster strains possessing a mutated n-acetylcholine esterase receptor subunit have been shown to high resistance to insecticides (Perry et al., 2008; Watson et al., 2010). Such resistance can be attributed to the changes in proteins targeted by insecticides (target site mediated resistance), increased rate of insecticide bio degradation (metabolic resistance), altered transport through the gut. (Hemingway & Ranson, 2000; Hemingway, Hawkes, Mccarroll, & Ranson, 2004; Nkya, Akhouayri, Kisinza, & David, 2013).
This information initiates our interest in testing the potency of multiple emulsions on the mortality of Drosophila virils and the ability of the fly to ingest and excrete the emulsions.
Materials and Methods
Insects D. virilis were maintained on a standard Drosophila diet (oatmeal, 7.5 %; molasses, 5 %; agar, 8.4 %; yeast, 8.4 %; methyl paraben, 0.35 % in water) at 25 o 96 C in a 12:12 light-dark cycle. Third larval instar were used in this study for dissections due to their size.
Materials
The pH-sensitive dyes, bromocresol purple and bromophenol blue (Sigma Aldrich) were used to determine intestine pH. To investigate the particle size ingestion limit of the pest, methyl methacrylate (MMA, ≥ 99 % Sigma Aldrich) was selected as the non-responsive monomer (purified using basic alumina) to produce particles via suspension polymerization. 2, 2-Azobis(2-methylpropionitrile) (AIBN, DuPont chemicals) was used as the radical initiator for polymerization. Sudan III (≥ 85 %, Sigma Aldrich) was chosen as a lipophilic dye to stain the particles for easy detection.
To form the oil-in-water emulsion precursor, the hydrophilic emulsifier, poly(vinyl alcohol) (PVA, ≥ 99 %, Mw 65,000, Sigma Aldrich) was utilized. FITC-dextran (average Mw 10,000, Sigma Aldrich) was chosen as the model active ingredient as it was suited to monitor the location of the species to encapsulate via fluorescence/confocal microscopy.
The pH-responsive monomer 2-vinylpyridine (2VP, 97 %, Sigma Aldrich) and non-responsive monomer methyl methacrylate (MMA, ≥ 99 % Sigma Aldrich) were purified using basic alumina. The oil phase consisted of the monomer; the primary emulsion surfactant stabilizers Span 80 (Sigma Aldrich) was used. The hydrophilic secondary emulsion polymer stabilizer, poly(vinyl alcohol) (PVA, ≥ 99 %, Mw 65,000, Sigma Aldrich) was used. Milli-Q water was used at all times. Resulting particles were cleaned to remove any unreacted monomer by multiple centrifugation and re-dispersion steps. Particle Ingestion Colored particles were fed to larvae. The particles were mixed with the standard Drosophila diet, and the flies were kept on this diet in a plastic petri dish before observations were made. After two days of feeding, the whole intestine was dissected in water and images were taken by optical microscopy (Leica m165fc). The dispersed particles were then observed by optical microscopy (Olympus BX51).
Mortality of the larvae after using the PMMA
2ml 30% W/W capsule solution was centrifuged; 0.5 ml water was removed to be more concentrated, vortexed, and re suspended. 0.5 ml was taken. This process was repeated three times to get a final concentration of 72.6 %. Percentage mortality of 3rd larval instar of the of D. virilis after treated with different concentration of microcapsules extract for 24, and 48 hours were calculated.
Results and Discussion
Con. |
Con. * 1 |
Log (Con. * 1) |
Treated |
Observed response % |
Linear response % |
Linear probit |
---|---|---|---|---|---|---|
30 |
30 |
1.4771 |
15 |
6.667 |
13.0906 |
3.8778 |
39.4 |
39.4 |
1.5955 |
15 |
26.666 |
22.1784 |
4.2338 |
43.8 |
43.8 |
1.6415 |
15 |
33.333 |
26.5036 |
4.3721 |
72.6 |
72.6 |
1.8609 |
15 |
46.667 |
51.2633 |
5.0317 |
LC% |
Con. mg / ml |
---|---|
25 |
42.268 |
50 |
70.8519 |
75 |
118.7659 |
90 |
189.0665 |
95 |
249.7174 |
99 |
420.8078 |
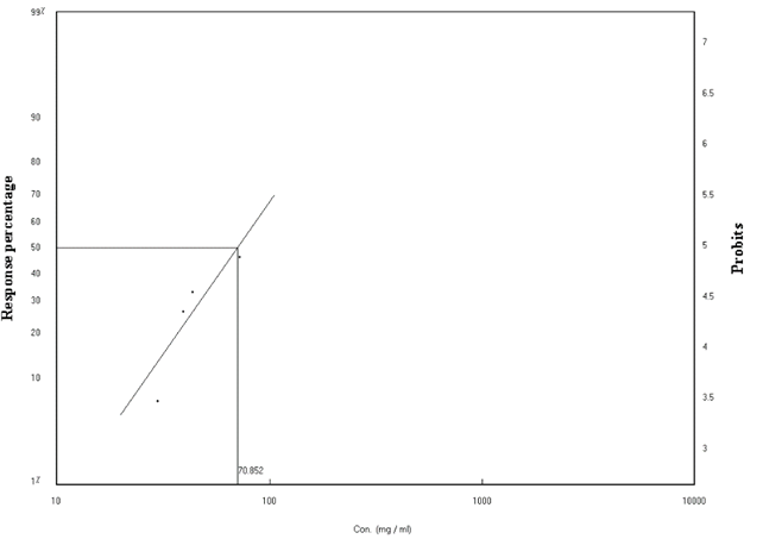
Con. |
Con. * 1 |
Log (Con. * 1) |
Treated |
Observed response % |
Linear response % |
Linear probit |
---|---|---|---|---|---|---|
15 |
15 |
1.1761 |
15 |
26.666 |
27.9333 |
4.4152 |
22.5 |
22.5 |
1.3522 |
15 |
40 |
34.9349 |
4.6129 |
30 |
30 |
1.4771 |
15 |
40 |
40.2511 |
4.7532 |
39.4 |
39.4 |
1.5955 |
15 |
40 |
45.4671 |
4.8861 |
43.8 |
43.8 |
1.6415 |
15 |
46.667 |
47.5207 |
4.9378 |
72.6 |
72.6 |
1.8609 |
15 |
60 |
57.3031 |
5.1841 |
LC% |
Con. mg / l |
---|---|
25 |
12.4798 |
50 |
49.7639 |
75 |
198.4362 |
90 |
689.1299 |
95 |
1451.624 |
99 |
5870.914 |
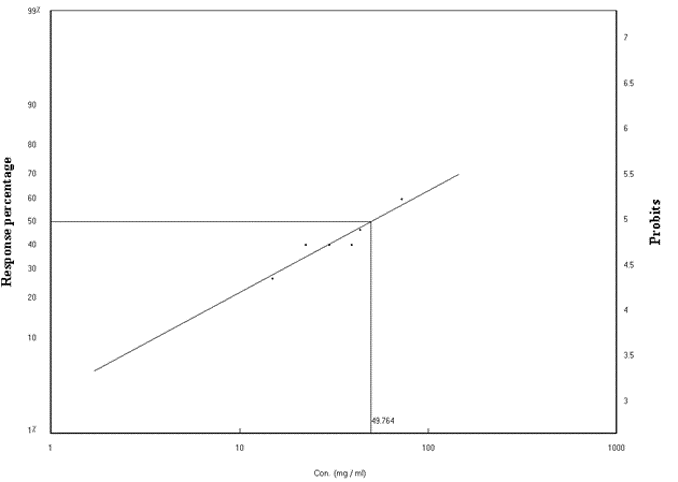
No |
Line name |
LC50 |
Index |
RR |
Slope |
LC50 |
LC90 |
---|---|---|---|---|---|---|---|
1 |
48 hrs. |
49.764 |
100 |
1 |
1.123 |
49.764 |
689.13 |
2 |
24 hrs. |
70.852 |
70.237 |
1.424 |
3.007 |
70.852 |
189.066 |
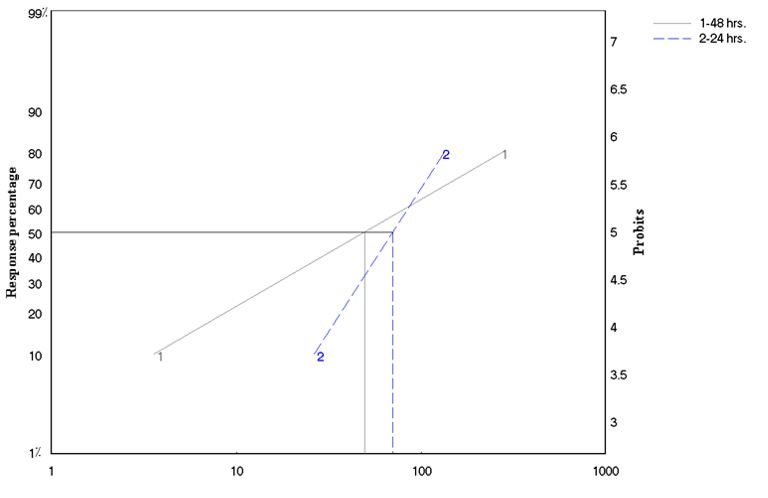
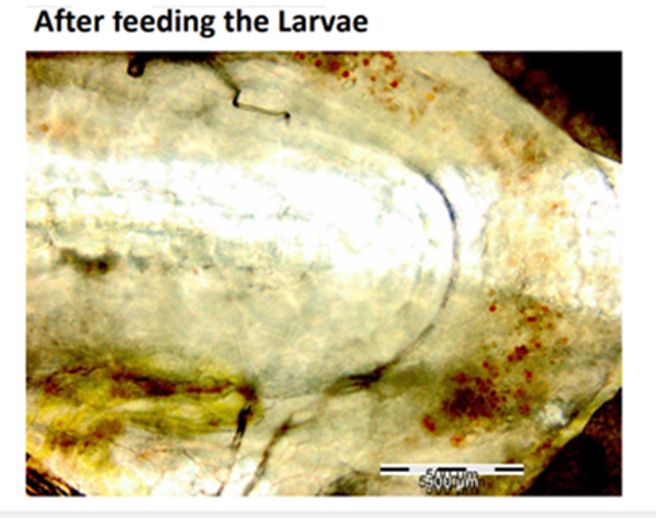
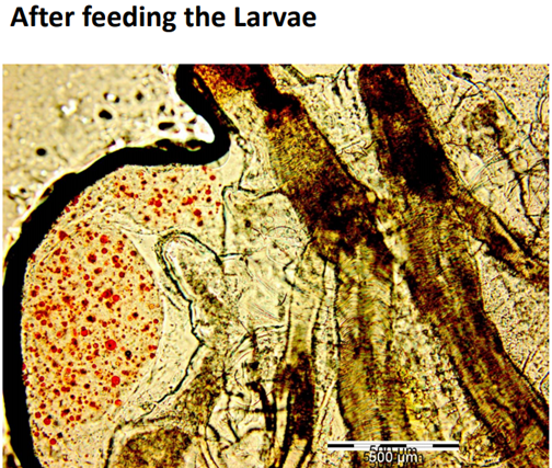
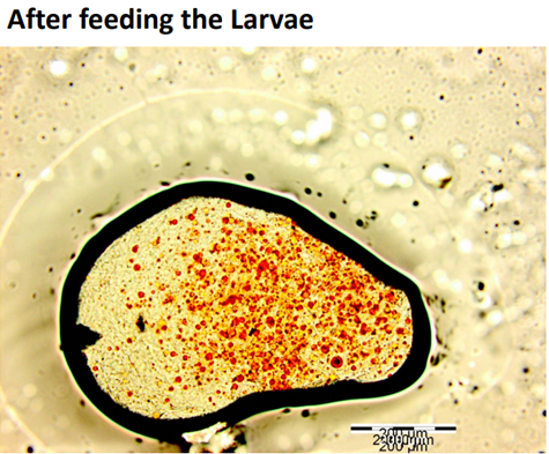
As safety measures, both the nature of the pesticide, including its formulation and the proposed method of application must be taken into account. To establish the effects of the formulation on D. virilis in vivo, we examined the dose-response of drug treatment on survival in 3rd instar larvae.Table 1 demonstrates the mortality rate of the 3rd instar larvae after treatment with different concentrations range between 30.00 - 72.6 mg / l. 24 hours of treatment induces % mortality ranging from (6.66 to 46.66%). The regression line representing the relationship between mortality ratios for the 3rd instar larvae of the D. virilis insect and the concentrations of the formulation (Figure 1) and the line of regression (Lc-p line) were determined. The concentration value needed to kill 50% of the treated larvae (LC50) (70.85 mg / l) and the slope of the regression line (1.272 ± 3.0066) was calculated. This concentration is a little bit higher than that of Cisplatin as a potent drug that induces a significant decrease in survival of 50% in larvae and 77% in adult D. virilis when 50 mg/l was used (Podratz et al., 2017). The values of Chi (data) Calculated (1.209) is lower than the tabular (6.00) at degrees of freedom n-2), indicating that there is homogeneity in the ratios of death obtained. The table also shows that there is a strong correlation between the concentration and the death rate (r) Correlation coefficient (0.95). Confidence limits for LC50 were calculated according to the (Litchfield & Wilcoxon, 1949) method for statistical analysis. The mean concentrations of 25%, 75%, 90%, 95% and 99% were also calculated as shown in Table 2.
The death rates ranged from 26.66 to 60.00% (Table 3). The regression line representing the relationship between mortality ratios for the third larvae of the D. virilis insect and the concentrations of the formulation (Figure 2), 48 hours later, and from the line of regression (Lc-p line), the concentration value needed to kill 50% (LC50), which is 70.851mg / l) and the gradient line slope (0.6254 ± 1.1228) was calculated. The Chi values of the Factual data and the tabular value were also calculated. The calculated Chi value (0.4116) When the third-year larvae are exposed to the same concentrations for a period of 9.5) at degrees of freedom n-2). This shows that there is homogeneity in the proportions of death obtained. The Table 5 also shows that there is a strong correlation between the concentration and the death rate (r) Correlation coefficient (0.9442). Confidence limits for LC50 were calculated according to the (Litchfield & Wilcoxon, 1949) method for statistical analysis. The mean concentrations of 25%, 75%, 90%, 95% and 99% were also calculated as shown in Table 4.
Table 5 demonstrates the effect of treatment duration on LC50 and LC90. It can easily be noticed that while the LC50 was only 1.42 folds higher after 48 hours compared to that after 24 hours, the LC90 was 3.65 folds higher.
Taking into account the role of the duration of exposure in the effectiveness of the product and its impact on the effectiveness of toxic effect, the study showed that the value of the lethal concentration of half the number of individuals decreases when the exposure duration increases. The obtained results show the sensitivity of the larvae to the toxic effect of the product. This can help to suggest that this type of material can be more effective and lasting effect for as long as possible.
It is well accepted that the digestive tract has a critical role in the digestion and absorption of different nutrients. Drosophila midgut harbors a chitinous matrix called the peritrophic membrane, which wraps around the ingested food and protects the epithelium from mechanical, chemical, and microbial insults (Lemaitre & Miguel-Aliaga, 2013), and the presence of midgut acidic region acts as a biological barrier shaping accumulation and distribution of ingested materials. Based on this Figure 6; Figure 5; Figure 4 which demonstrate the ingestion and excretion of the formulated particle in the hind-gut can help to suggest that our formulation was able to pass through the peritrophic membrane and the midgut acidic regions as two barriers (Storelli et al., 2018).
In conclusion, we found that the prepared microcapsule have insecticidal potency against D. virilis in a dose-dependent homogenous pattern. This can be of the critical need to avoid the developed resistance to commonly used insecticides. Further, studies on the mode of action of the prepared microcapsule is critically needed.
Conclusion
In conclusion, the formulated micro capsule of the multiple emulsions has a satisfactory insecticidal potency against Drosophila virilis in a dose-dependent manner which might help to avoid the developed resistance to commonly used insecticides.