Stabilizing effect of chitosan-poly vinyl alcohol complex on simvastatin loaded nanoformulation
Abstract
Biopolymers are attractive new and general means of presenting more stable drug delivery system. This is accomplished by encapsulation of polymers to respective drug molecules which offer formation of bonds between them. In this work, chitosan-coated simvastatin nanoformulation was formulated using a method named solvent evaporation technique, and various stability studies were performed. The steadiness of preparation was evaluated by accelerated stability method and forced degradation method. High-performance thin-layer chromatography (HPTLC) was utilized to detect the degradant present in formulation after subjecting it in different stress condition. Simulated annealing process for the prediction of nanoformulation stability was also performed. The accelerated stability study was performed at three altered temperature conditions, (i.e.) accelerated temperature (40 ± 2 °C/75 ± 5% RH), room temperature (25 ± 2 °C/60%) ± 5% RH, and freezing temperature (4°C) and change in drug content, solubility, % cumulative drug release, moisture content and molecular weight were calculated. Standard sample and nanoformulation were exposed to different stress conditions (thermal, base, acid, and light). Finally, the percentage of decomposition was calculated by HPTLC method. All stability studies, along with the simulation method, represented the stability of simvastatin in nanoformulation. Establishment of adequate shelf life also was achieved. This work evidenced the extensive stability offered by chitosan polymers to the prodrug simvastatin. Also, give insight when the problem of poor stability of drug molecules limits its practical applicability.
Keywords
Simvastatin, Chitosan, Poly vinyl alcohol, HPTLC, Stability, Accelerated stability
Introduction
At the present time, newer drug developments, and the progress of various innovative drug delivery system has to turn into a modern perception in the pharmaceutical field. Many polymers with a capacity of decreasing frequency of dose, increasing drug’s efficacy and delivering the drug at the particular region seem to be favorable as drug cargo. Amongst numerous polymers, a natural polysaccharides-chitosan owing to its mucoadhesiveness and biocompatibility is considered for pharmaceutical purposes including sustained or smart drug delivery system. Regardless of the statement that chitosan is a distinctive and multipurpose polymer, utilized extensively in the various discipline, it has foremost drawback which restricts its use due to its instability at that condition.
One is its biological activity appears only in acidic medium and at acidic pH, amino groups which is free, undergo ionization leading to electrostatic repulsion within the polymer chain. This permit polymer solubility. Sometimes it degrades at the extreme acidic condition. Another is it is stable in all stress condition except in UV irradiation. UV irradiation modifies its surface characteristics leading to instability (Mucha & Pawlak, 2002). But any intermolecular bonding achieved with other polymer or with small molecular weight compound may modify this properties and enhance its stability.
The drug molecule, simvastatin used in this research, is a prodrug and unstable in the GIT and metabolized extensively at the gut and liver. Due to this reason its active moiety lactone ring-opened in GIT (which should be opened in the liver to produce respective pharmacological action) leading to poor absorption, in parallel less bioavailability. Simvastatin degrades significantly in all stress conditions and to some extent, by light-induced stress. The simvastatin extensively degrade at the alkaline condition.
Stability testing is the crucial section in the pharmaceutical platform for a candidate either it is a new drug nor a new formulation (Bakshi & Singh, 2002; Committee for Proprietary Medicinal Products (CPMP), 2002; International Conference on Harmonization (ICH), 2003; Strasbourg, 2007; W.H.O, 2007). In general active pharmaceutical ingredient (API) present in dosage forms undergo degradation upon storage. Most of the industries carry out forced-degradation studies (stress testing) in pilot plant scale before the commencement of bulk formulation which would be useful in the selection of excipients for further steps (Krishnamurthy & Manning, 2002). This enable optimized trials for developing analytical methods for identifying stability. As evidenced by many researches, there are various analytical methods to identify the simvastatin in biological samples. However, the presence of degradants of simvastatin in drug delivery systems needs to be carried out. Currently, HPTLC is an emerging analytical tool since it can be operated with low cost and require minimal quantity of samples and many samples could be analyzed at a time (Sethi, 1996; Wu, 2000).
In this work, the nanoformulation of simvastatin encapsulated with chitosan presented modified drug properties due to the protection in GIT leading to enhanced biological properties which was presented in our previous paper (Selvasudha & Koumaravelou, 2017). This effect was observed due to the stability offered by chitosan, biopolymer. The intermolecular hydrogen bonding formed between simvastatin and chitosan, inter complex network between chitosan and PVA (stabilizer used in nanoformulation) not only stabilize chitosan in acidic medium but also make chitosan to impart its stabilizing effect to the simvastatin which was proved and discussed in the present paper.
Materials and Methods
API-Simvastatin was obtained from Biocon Pvt. Ltd. All solvents of analytical grade and polymers viz. Polyvinyl alcohol, medium molecular weight chitosan (400KDa), and surfactant polysorbate 80 (Tween 80) were purchased from Sigma Aldrich.
Preparation methods
Emulsion solvent evaporation technique was selected for the nanoformulation of simvastatin. Methanol is used to solubilize the simvastatin (organic phase). 0.5% polyvinyl alcohol and 0.2% tween 80 in water along with chitosan in 2% acetic acid constituted aqueous phase. The organic phase was added slowly to the aqueous solution under magnetic stirring. The rpm maintained was 400 for the time period of 3h. Further homogenization was carried out at 10,000 rpm with the help of overhead stirrer after addition of sucrose as lyoprotectant. Nanoparticles were detached from the supernatant with the help of cooling centrifuge adapting ideal conditions (20,000 rpm for 30 min) followed by lyophilization. The basic nanoparticle characterization were explained in our previous paper (Selvasudha & Koumaravelou, 2017).
Characterization
Nanoparticle characterization
The prepared formulation was evaluated for its morphology using optical microscopy (Nikon polarizing microscope) and also for % encapsulation efficiency and drug loading as per the procedure described by Selvasudha and Koumaravelou (2017).
Simulated Annealing Method for the Prediction of Nanoformulation Stability
Preparation of Biopolymer and Ligand
3- D structures of Chitosan retrieved from Chemspider (64870) and Simvastatin retrieved from Pubchem (54454). SYBYL-X 1.3 was used for structure preparation. Bond orders assignment and Restrained impref minimization were carried out. The final structure was utilized further.
Molecular docking
Dock suite program in the SYBYL-X 1.3 was one used for this research studies. Hierarchical series of filters and Induced Fit docking (IFD) protocol were adapted, and studies were carried out according to Balasubramaniyan, Irfan, Umamaheswari, and Puratchikody (2018).
Annealing
Molecular dynamics involving simulated annealing method in which thermal energy of the complex model of Simvastatin with Chitosan is cycled over time. Temperature (40oC) along with the charges Gasteiger-Marsili to surmount torsional barriers; energy is then removed by lowering the temperature to obtain a low-energy structure.
Accelerated Stability Study
Estimation of Nanoformulation Properties
The specification mentioned in the ICH guideline Q1A was followed to determine the stability of the nanoformulations. Using stability chamber, the studies were performed at the conditions of accelerated temperature (40 ± 2 °C/75 ± 5% RH), room temperature (25 ± 2 °C/60%) ± 5% RH, and freezing temperature (4°C). The samples before and after stability studies were analyzed for solubility, drug content, % cumulative release, moisture content and molecular weight. For this samples were withdrawn from 0 months up to 6 months at every three months period to find out the effect of humidity and temperature on nanoformulation stability. In addition, the samples were subjected to FT-IR analysis to confirm the stability of nanoformulation.
Drug Content Determination
Nanoparticles weighing about 10 mg has been added to 10 ml of methanol. This solution was passed through a 0.45µm syringe filter after sonication for 3 min (Padhye & Nagarsenker, 2013). Finally, using UV/visible spectrophotometer at 238 nm, the drug content was calculated.
Saturation Solubility Studies
Saturation solubility measurement for nanoformulation has been performed as per the procedure of (Soni, Dandagi, Gadad, & Mastiholimath, 2011). The surplus amount of formulation was filled in the 10 mL container, which already contained 5 mL of the buffer with pH 5.5. The vials were shaken in an orbital shaker for 12 h at 25ºC. The solutions were allowed to stand for another 24 h. The supernatants were analyzed for drug content on UV–Visible spectrophotometer (Labindia 2000) at 238 nm after filtration and proper dilution.
In-Vitro Release Study of Nanoformulation
Calculated quantity of nanoparticles were dispersed in the acetate buffer pH 5.5. This was taken in a dialysis membrane bag with a molecular weight cut-off of 12 KDa and tied. The membrane was placed in a conical flask which had 100 ml of the same buffer. The set up was kept in an orbital shaker for 8h at 50 rpm and at room temperature. The required samples were withdrawn at a specified interval of time and replenished with fresh buffer (Tatiya & Basarkar, 2013). The free drug concentration was evaluated using UV–vis spectrophotometer at 238 nm and calibration curve method was adapted for calculation.
Moisture Content
Gravimetric method (Black, 1965) was used to determine the moisture content of the formulation. The sample was dried every 1h up until constant weight attainment, and samples were weighed before and after drying. Percentage of moisture content was calculated using formula.
% of moisture content =
Viscosity Average Molecular Weight Method
Ubbelohde capillary viscometer (Schott Gerate, Germany) was used to calculate the intrinsic viscosity of nanoformulation. Calculated quantity of nanoformulation and chitosan were solubilized in 2% glacial acetic acid. The viscosity average molecular weight was calculated according to the Mark-Houwink equation, using intrinsic viscosity (where K = 0.119, a = 0.59).
Fourier transform infrared (FT-IR)
The structural features of nanoformulation before and after stability studies were analyzed using FTIR (Fourier transform infrared) (FTIR- 410®Jasco Colchester, UK) by KBr pellet method.
Shelf-life determination
Q 10 method was followed to determine the shelf life of formulation, where Q 10 is the factor in which rate constant is directly proportional to 10°C rise in temperature. The absorbance of simvastatin in the formulation (in methanol) was analyzed at 238nm using UV-Vis spectrophotometer. The equation used for the calculations are
Where A is the pre-exponential factor, Ea is activation energy, R is the universal gas constant, and T is the temperature in degrees Kelvin.
Where Co is initial concentration, K is rate constant.
High-Performance Thin Layer Chromatography (HPTLC)
Instrumentation
The samples were applied at the width of 6mm with a Camag 100μL syringe (Hamilton, Bonaduz, Switzerland) on silica gel precoated aluminum plate 60 F – 254, (10cm×10 cm) with 250 μm thickness; Merck, Germany, using a CamagLinomatV (Switzerland) sample applicator. Methanol has been used for prewashing. Scanning speed, slit dimension and monochromator bandwidth were maintained at 4.0 mm/s, 4×3 mm and 10nm respectively. Mobile phase combination of Chloroform and Methanol in the ratio of 9.5: 0.5v/v was finalized, and the experiment was proceeding with all other general instruction followed for HPTLC. After completion, the plates were dried with air dryer. Camag TLC scanner II in the absorbance-reflectance mode at 254 nm using CATS software was used for densitometric scanning. The concentrations of the compound chromatographed were determined using densitogram area.
Standard Solution Preparation
Calculated quantity of sample was taken in the 100 mL flasks and dissolved in methanol. The volume was made up to 100 mL with methanol itself. From this 10 mL was pipetted out into a 50 mL volumetric flask and made up the volume with methanol to give a concentration of 200 μg.mL−1 (1000 ng.μL−1).
Sample Solution Preparation
Calculated quantity of sample was taken in the 100 mL flasks and dissolved in methanol. The volume was made up to 100 mL with methanol itself after sonication for about 15 min. Filtration was performed using Whatman filter paper No.41. 10 mL of the solution is pipetted out into a 50 mL flask and made up to 50 mL with methanol. From the resulting solution, 5μL was spotted, and chromatoplate was developed. After development, the bands of the sample were scanned at 238 nm using a densitometer. The peak area of standard and sample were used to calculate the amount of simvastatin and present and corresponding degradation.
Forced Degradation Studies Using HPTLC
Through this study, the stability of chitosan entrapped simvastatin nanoformulation was analyzed, and possible degradation products were identified as per the procedure described by (Selvasudha, Koumaravelou, & Durgadevi, 2017). The samples were subjected to acid, base, oxidation, thermal, and photodegradation studies and results were evaluated.
Results and Discussion
The size of particles was found to be nanosize with spherical morphology (Figure 1). The % entrapment (encapsulation) efficiency and % drug loading were calculated to be 98.2±0.007%, and 17.0±0.11% In our previous study (Selvasudha & Koumaravelou, 2017a) the nanoformulation prepared using 1:5 simvastatin to chitosan ratio, 0.5% PVA and 0.02% Tween 80 emphasized upon modified and improved simvastatin poor physicochemical properties because of modified and inherent characteristics of chitosan. The improved pharmacological activity also reported owing to the beneficial chitosan and simvastatin synergistic interaction. The basis for these altered properties was found to be stability offered by chitosan and chemical bond formed in between simvastatin and chitosan during the formulation process. This paper discuss the results of various stability studies and factors responsible for the formation of this stable simvastatin and chitosan nanocomplex.
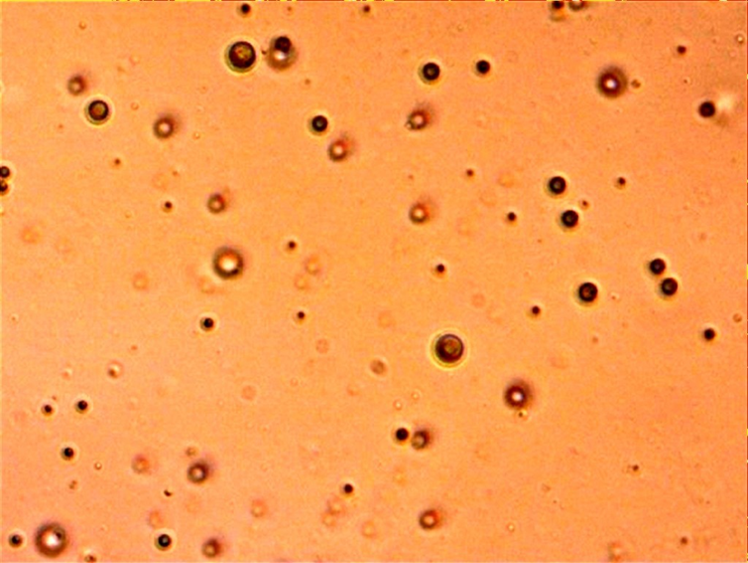
Simulated Annealing Method for the Prediction of Nanoformulation Stability
The three-dimensional structures of Chitosan and Simvastatin reclaimed using the Chemical Data Bank are shown in Figure 2. Chitosan and Simvastatin were solved and the atom of Chitosan almost
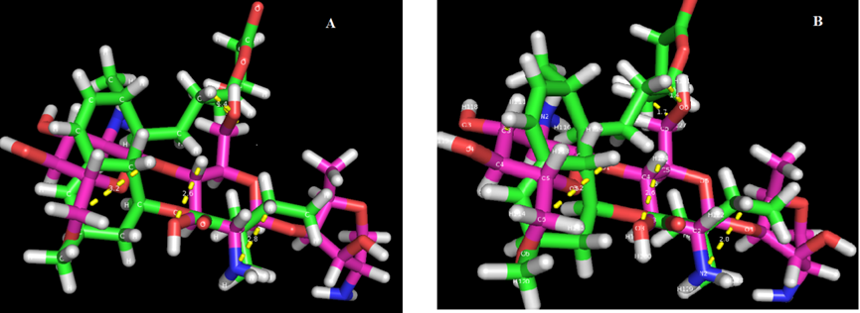
all interacts with simvastatin. The simvastatin, which is considered as co-crystallized ligands, interact through a hydrogen bond with the atom NH2, C2, C6, O6, O3 of chitosan. The simvastatin-ligand was additionally strengthened by more number of hydrophobic groups with other atoms. The Chitosan and Simvastatin interaction total score is 6.56 and the ligand atom form hydrogen bond with the atom present in Chitosan used to be more than 6 with below 3Å bond length. Evaluation of the energy profile of Chitosan with Simvastatin activity showed the binding mode and interaction pattern, and it proves that chitosan increase the solubility and absorption of Ligand. Stability of Simvastatin sustains till 40oC (Figure 2).
Accelerated Stability Studies
Estimation of nanoformulation properties
The result (Table 1) represents insignificant changes in appearance and also in solubility, drug content, % cumulative simvastatin release from formulation, moisture content, and molecular weight at room temperature condition. At the storage condition mentioned in the methodology section, the solubility was found to be 2.18± 0.40µg/mL, indicating no greater effect on solubility after six months of stability study (Table 2). The drug content was found to be 122±7.0µg/5mg of nanoformulation, representing a negligible difference from the sample before storage. Cumulative percentage of Simvastatin from nanoformulation was 60.34±0.0 % at the end of 6 months, indicating the change was within the limit. The moisture content and molecular weight also decreased but ranged within the acceptable limit. The characteristics of formulation at refrigeration temperature also found to have a negligible difference (Table 3). Moreover, all characteristic FT-IR peaks of formulation, i.e. 3417 cm−1, 2925.8 cm−1 and at around 1770 cm−1 which split into three segments (1699 cm−1, 1710 cm−1, 1724 cm−1) were retained after 6 months in all temperature conditions (Figure 3). Hence, the nanoformulations are stable for a requisite time period at different storage conditions.
Test |
0 months |
3 months |
6 months |
---|---|---|---|
Drug content µg/5mg |
134 ± 10.20 |
132 ±4.0 |
132 ± 5.2 |
Solubility µg/mL at pH 5.5 |
2.31 ± 0.92 |
2.31 ± 1.2 |
2.30 ± 1.4 |
% Cumulative drug release |
66.18 ± 1.26 |
66.34 ± 3.2 |
65.7 ± 0.8 |
Moisture content % |
2.7 ± 0.03 |
2.9 ± 0.01 |
2.9 ± 0.07 |
Molecular weight Da |
70,000 ± 10,000 |
70,000 ± 10,000 |
70,000 ± 10,000 |
Test |
0 months |
3 months |
6 months |
---|---|---|---|
Drug content µg/5mg |
134 ± 10.2 |
129 ± 5.8 |
122 ± 7.0 |
Solubility µg/mL at pH 5.5 |
2.31 ± 0.92 |
2.01 ± 0.45 |
1.98 ± 0.80 |
% Cumulative drug release |
66.18 ± 1.26 |
62.34 ± 5.8 |
60.34 ± 0.0 |
Moisture content % |
2.7 ± 0.03 |
3.4 ± 0.05 |
3.5 ± 0.01 |
Molecular weight Da |
70,000 ± 10,000 |
67,000 ± 8,000 |
67,000 ± 8,500 |
Test |
0 months |
3 months |
6 months |
---|---|---|---|
Drug content µg/5mg |
134 ± 10.2 |
131 ± 3.4 |
130 ± 6.3 |
Solubility µg/mL at pH 5.5 |
2.31 ± 0.92 |
2.2 ± 0.21 |
2.18 ± 0.40 |
% Cumulative drug release |
66.18 ± 1.26 |
65.56 ± 2.2 |
65.34 ± 1.92 |
Moisture content% |
2.7 ± 0.03 |
2.9 ± 0.03 |
2.9 ± 0.05 |
Molecular weight Da |
70,000 ± 10,000 |
70,000 ± 10,000 |
70,000 ± 10,000 |
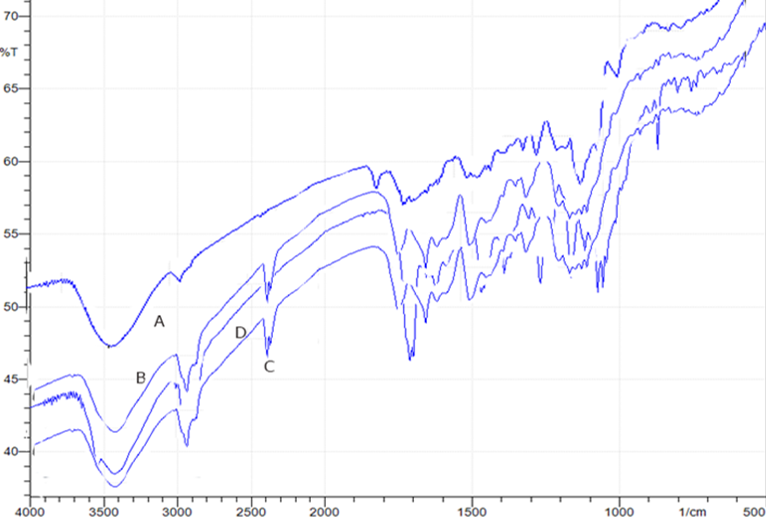
Shelf Life Determination
The shelf life at 25°C (room temperature) was calculated as 2.8 years. The chitosan encapsulated simvastatin nanoformulation stored at room temperature over 90 days exhibited good stability.
HPTLC
The peak observed for formulation at Rf 0.27, 0.43, 0.78 in all stress conditions represents the domination of polymer peak (Table 4) in nanoformulation parallely indicating entrapment. The peak observed for the pure drug (Table 5) with Rf 0.63, 0.68 disappeared, which represents the loading of simvastatin into the polymer. The peak at Rf 0.87 not found in both polymer and drug densitogram and it was confirmed that peak appeared due to the presence of Tween 80 surfactant by literature survey (Christiansen, Backensfled, Kohn, & Weitschies, 2011). From this, it is evident that polymer, surfactant protect drug core offering stability to the formulation (Table 6).
Stress applied |
Rf |
% Area |
---|---|---|
Acid induced |
0.63 |
75.15 |
Base induced |
0.64 |
72.20 |
Oxidative induced |
0.63 0.68 |
45.76 24.24 |
Light induced |
0.54 0.65 |
10.71 51.29 |
Heat induced |
0.64 |
85.00 |
Stress applied |
Rf |
% Area |
---|---|---|
Acid induced |
0.42 0.67 0.73 |
35.93 10.71 12.36 |
Base induced |
0.13 0.28 0.41 0.74 |
14.91 09.63 09.54 15.92 |
Oxidative induced |
0.27 0.39 0.41 0.74 |
12.91 12.39 14.49 10.26 |
Light induced |
0.14 0.18 0.28 0.40 0.42 0.73 |
19.30 30.97 09.50 11.09 14.14 15.00 |
Heat induced |
0.38 0.74 |
23.70 26.30 |
Stress applied |
Rf value |
% AUC |
---|---|---|
Acid induced |
0.27 0.78 0.87 |
11.66a 09.61a 78.74 b |
Base induced |
0.43 0.78 0.87 |
04.40 a 06.53a 89.08b |
Oxidative induced |
0.29 0.78 0.87 |
06.40a 05.40a 88.08b |
Light induced |
0.12 0.30 0.35 0.38 0.44 0.74 0.57 0.65 |
06.35a 28.79a 10.63a 09.51a 23.47a 17.47a 02.82c 00.97c |
Heat induced |
0.79 0.89 |
19.70a 80.20b |
a Corresponds to chitosan; b Corresponds to Tween 80; c Corresponds to Simvastatin
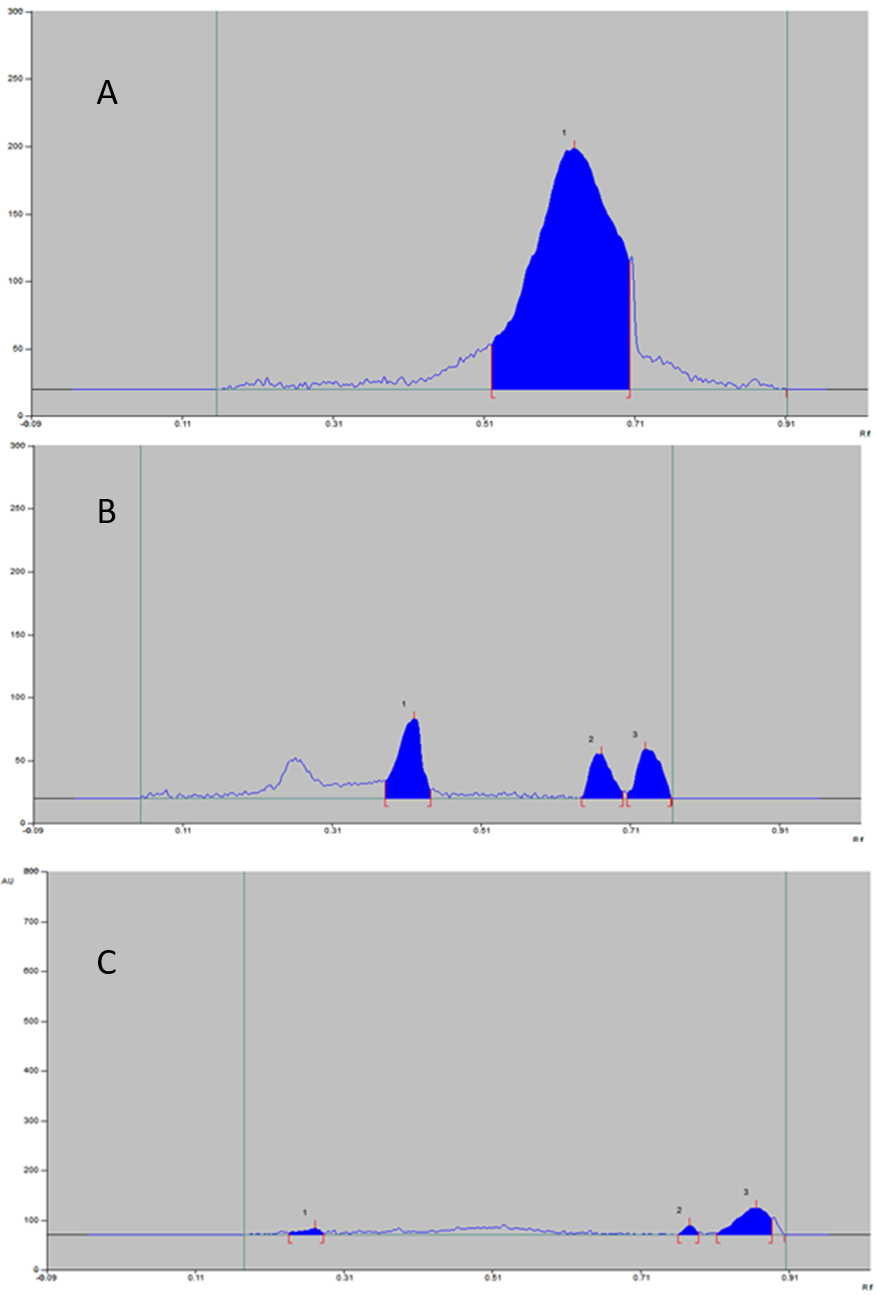
Acidic Stress-Induced Degradation Study
The nanoformulation was found to be stable in acid stress. The reaction in 0.001 M HCl at 40°C under reflex for 30 min showed no degradation products. Any additional peaks because of degradation products and even peaks of drug not viewed (Figure 4).
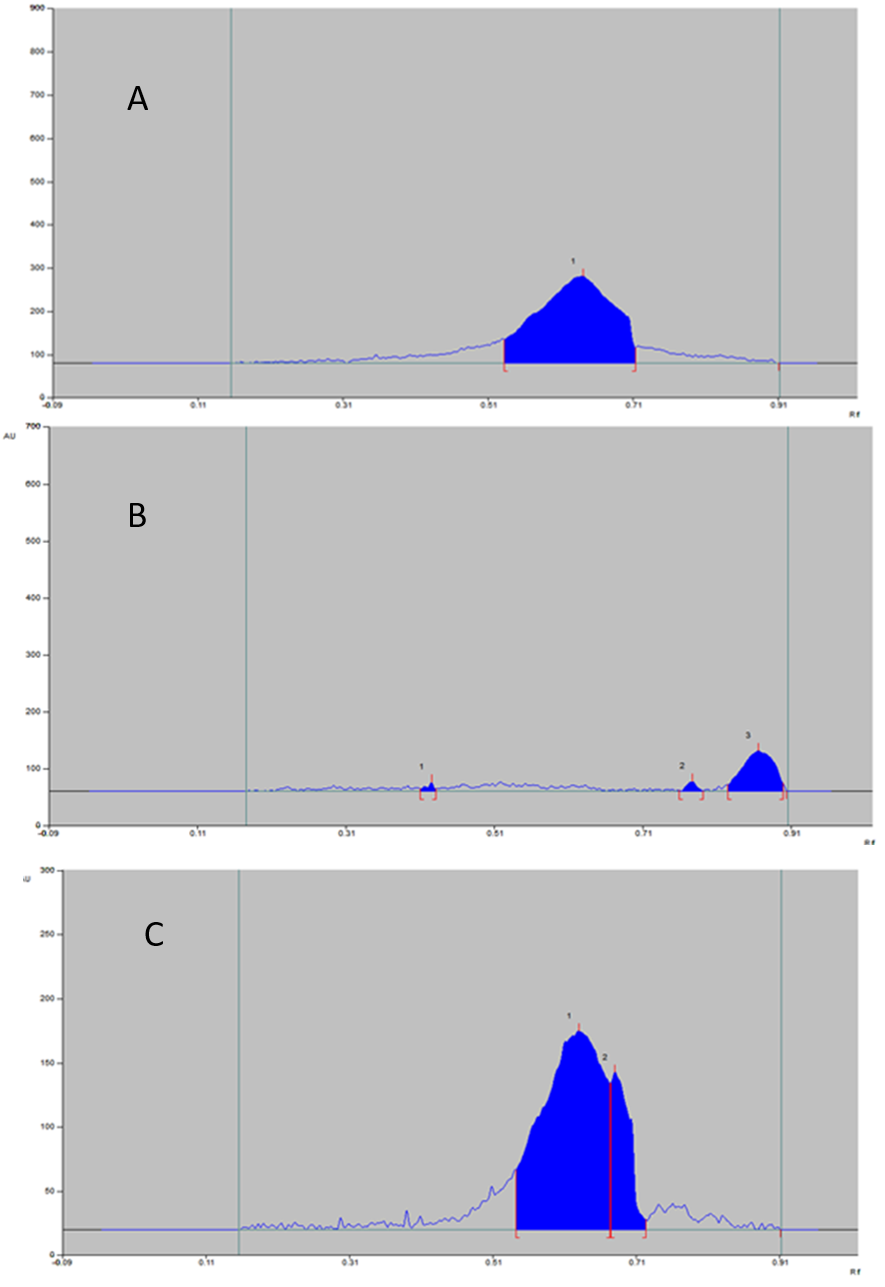
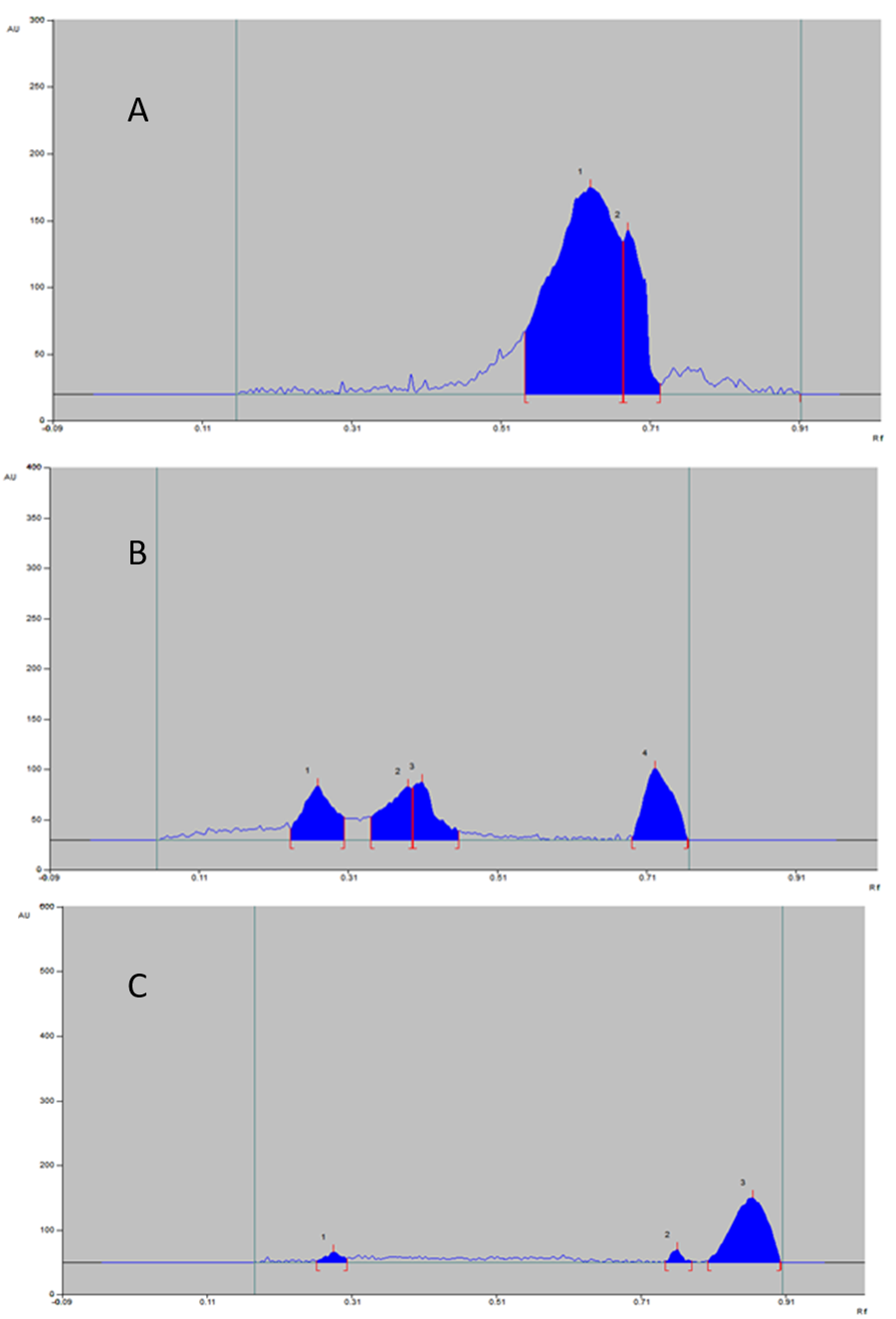
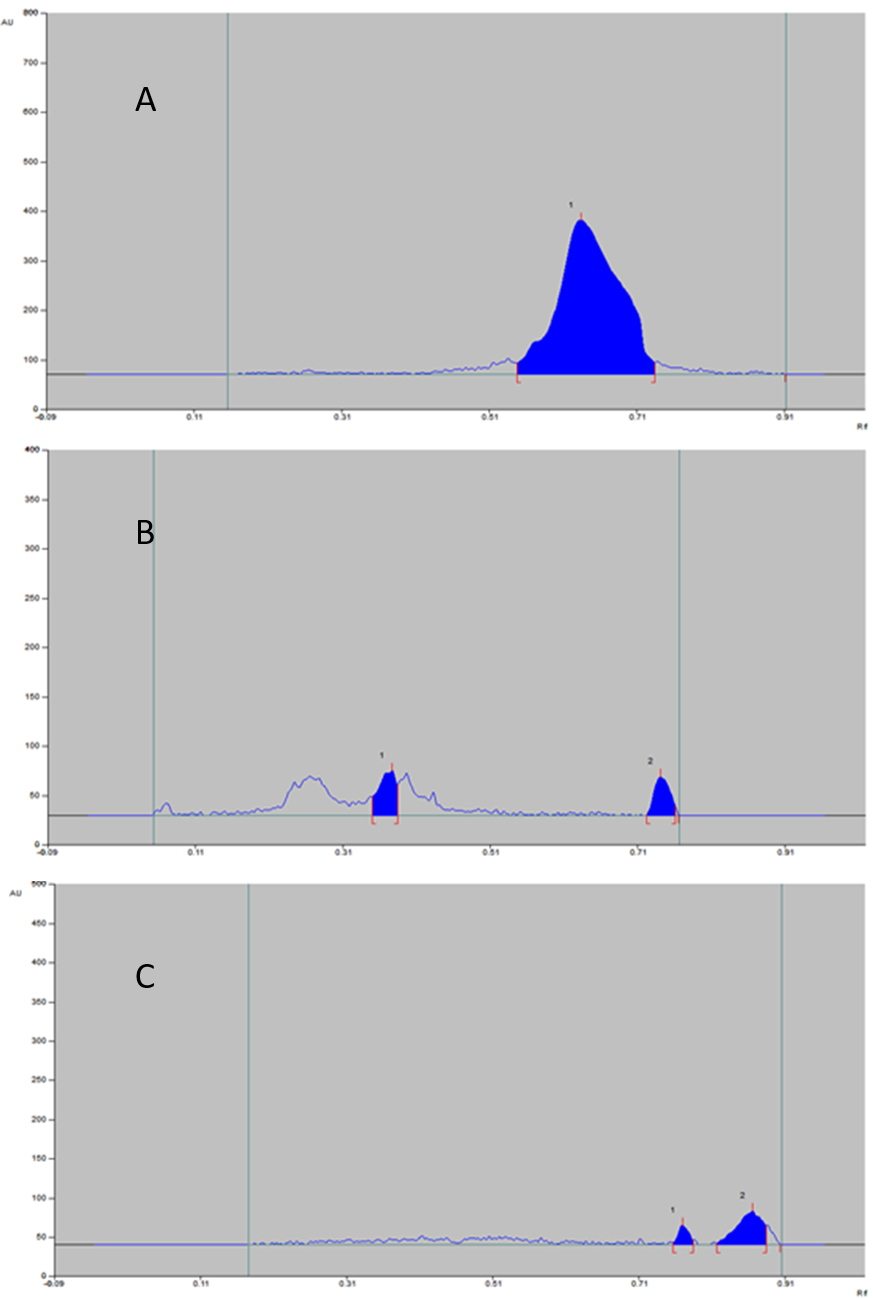
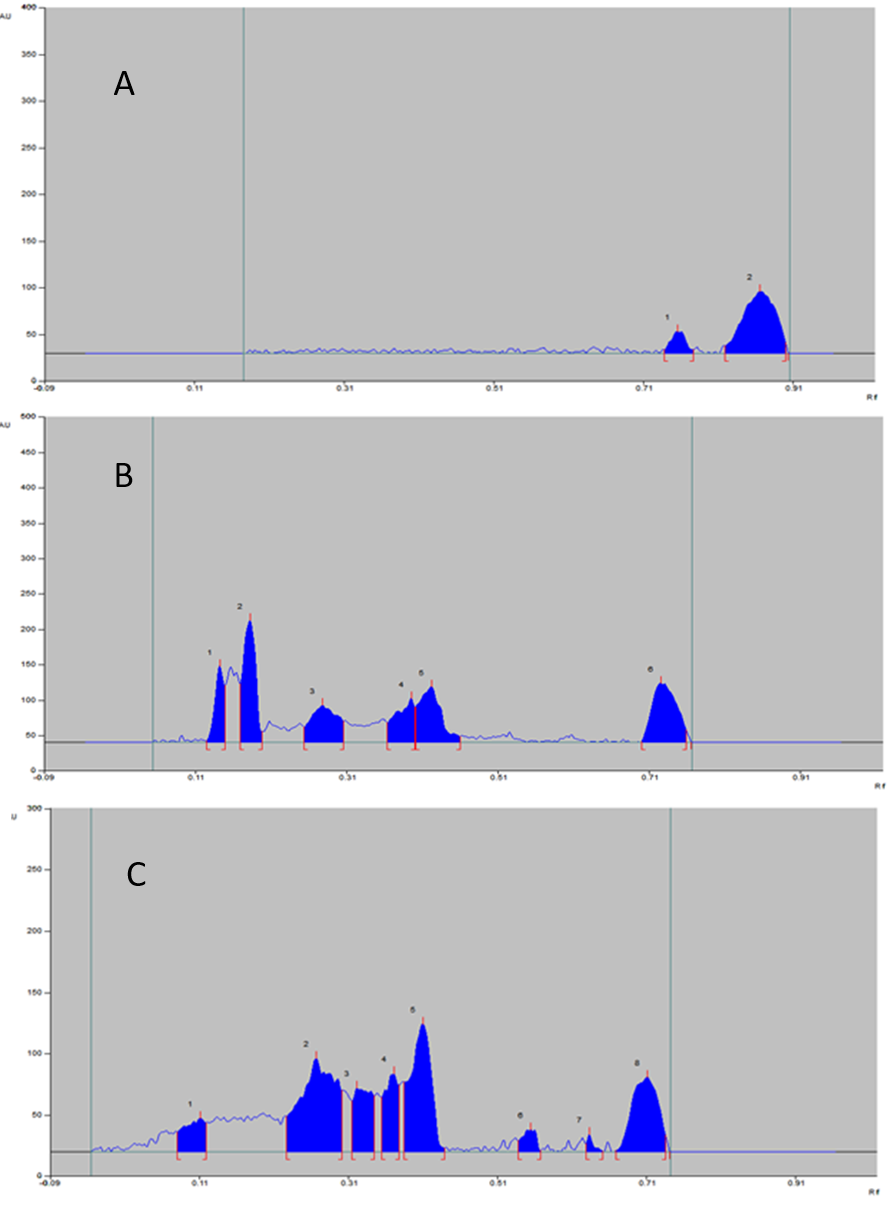
The percentage degradation of chitosan in the formulation also found to be very less and more percentage AUC observed for tween 80.
Basic Stress-Induced Degradation Study
The same results mentioned in acidic stress condition observed for basic stress condition (Figure 5). The percentage of degradation also very negligible as shown in Table 6.
Oxidative Stress-Prompted Study
In this study, it was seen that the formulation was not likely to decompose. The results revealed that the % degradation was negligible for formulation (Table 6). The densitogram for this study is displayed in (Figure 6). The reason attributed to this stability is antioxidant properties of both chitosan and simvastatin in addition to intermolecular hydrogen bonding.
Thermal (Heat) Degradation Study
The formulation was stable and % degradation was found to be inconsequential (Table 6) and Figure 7). The thermal protection given by the polymer is the reason for the stability of nanoformulation. In general, chitosan have melting endotherm at 215°C, but the melting endotherm of formulation converted to higher values. Therefore, at the heat applied during the study, the formulation won’t reach either glass transition temperature or melting endotherm of formulation offering stability (proved by DSC).
Photo (light) Degradation Study
The densitogram of formulation showed additional peaks as shown in Figure 7 representing degradation of chitosan in the formulation. Though the photostability is offered by chitosan to the formulation, more extensive protection is not possible. The chitosan is less stable in UV irradiation, but the simvastatin is stable in the same condition. The UV irradiation induces the free radical formation especially –OH radicals in chitosan which collide with each other forming extra crosslinks between chain producing new radicals. The degradation products of chitosan appeared in densitogram, but the peaks of simvastatin degradation products were not seen which indicate the stability of the drug in the formulation. The percentage area of the peak for pure simvastatin was also observed to be less. Nevertheless, production from light should be ensured.
The reason for stability of formulation is depicted in Figure 8. The many factors which influence the stability of chitosan may or may not affect the formulation stability, which will be discussed in further heads.
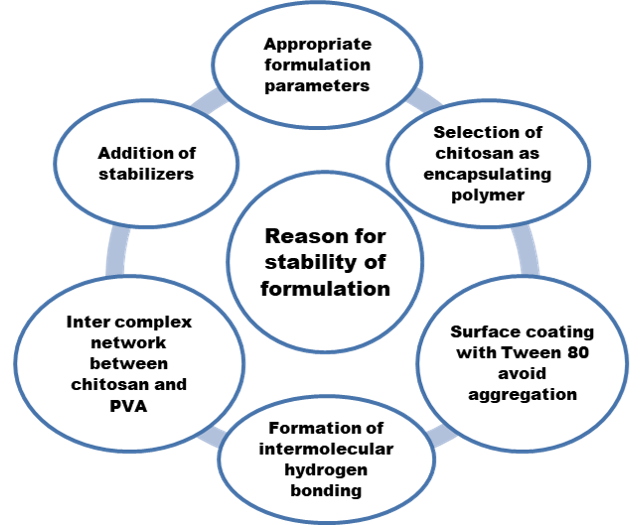
As simvastatin (active drug) used in this research for nanoformulation are less soluble in water and lipophilic in nature, the stabilizer PVA and surfactant Tween 80 incorporated during formulation to increase the wetting and dissolution properties of simvastatin (Choi, Yoo, Kwak, Nam, & Lee, 2005).
Steric stabilization created in nanoformulation offered by Tween 80-nonionic surfactants and PVA-polymer is based on the formation of a strong steric film, between the size reduced particles. The heat and pH changes, during the drying process, might hinder the movement of particles and therefore, the steadiness maintained in our study (Kim & Lee, 2010) leading to stability. The stabilization created by the combination of chitosan, PVA, drug and Tween 80 is created due to repulsive action between the ionized colloidal nanoparticles. The important factors for an efficient stabilization are strong entanglement to the solid surface, effective encapsulation of stabilizers on the nanoparticle and HLB balance of the stabilizer which are satisfactorily given by the appropriate concentration of stabilizers used in this formulation (Liu et al., 2015).
Role of Chitosan in Stability of Nanoformulation
The chitosan is an important material in the formulation since it encapsulate the active drug simvastatin. Therefore the parameters viz. molecular weight, deactylation degree, moisture content, concentration and pH of chitosan solution used in the formulation which affects the chitosan stability should be considered while formulation and also during storage (Szymanska & Winnicka, 2015).
Effect of Chitosan Concentration
Chitosan, a polysaccharide, possess a great affinity to negatively charged substances because inherently, it built with hydroxyl or amino groups which conveniently experience interaction (Burchard, 2001). The higher concentration of chitosan in nanoformulation makes it more prone for electrostatic bonding, which facilitate stability. This concentration-dependent stability offered by the assembly of thickened multi-sheath formation directly proportional to the charge of the polysaccharide [zeta potential (Zp)] (Carneiro-Da-Cunha et al., 2010). In general, greater positive or negative zeta potential (ZP) (between +30mV and −30 mV) offer the dispersion stability, and in parallel, it is understood that low Zp values lead to aggregation (Wongsagonsup, Shobsngob, Oonkhanond, & Varavinit, 2005). In this study, chitosan in formulation imparts positive Zp, +31.6 (data not shown) that inhibit aggregation leading to stability (Vargas, Albors, Chiralt, & Gonzalez-Martinez, 2009).
Effect of pH
pH reduction from 3.5 to 2.5 of formulation initiate a noteworthy rise in Z-average values consecutively increase in intrinsic viscosity, leading to chain extension of chitosan molecules (Pa & Yu, 2001). In common the acidic pH cause, protonation of –NH2 groups of chitosan producing –NH3 + groups. In formulation, the intermolecular hydrogen bonding between chitosan and simvastatin and PEC of chitosan and PVA resist the ionization of chitosan in an acid environment, decreasing the degradation, thereby increasing the stability of simvastatin in acidic pH.
Molecular Weight
The molecular weight (MW) is an important character of a polymer (chitosan), accountable for a number of its properties such as biodegradability, water-holding capacity, hydrophilicity, mucoadhesion, and viscosity. The thermal stability of chitosan depends upon its MW (Tsaih & Chen, 2003). Besides, a number of features viz. irradiation, strong acids, mechanical shearing, elevated temperature considered to impact the chitosan MW. For illustration, physical methods like overhead stirring, homogenization or centrifugation frequently used for the preparation of chitosan drug delivery system, were noticed to decrease the polymer MW (Dimonie, Dima, & Petrache, 2013). In this study, the conversion of medium molecular weight chitosan into low molecular weight has been noticed due to the above said physical methods used during the formulation process of nanoparticles. Anyhow, the formation of low M.wt chitosan did not exert any stress related to degradation during storage. The reason might be due to the stability offered by an intermolecular hydrogen bond between simvastatin and chitosan.
Degree of Deacetylation
More deacetylated chitosan, the slower will be the rate of acidic hydrolysis (Weinhold, Sauvageau, Kumirska, & Thöming, 2009). Medium molecular weight chitosan with 95% of DD used in this study has a less porous structure, that confines the faster degradation process in lower pH. Thermal depolymerization also inhibited to a certain extent due to crosslinking between chitosan and simvastatin/PVA, which exerts a stabilizing effect on the nanostructure.
Moisture Content
Chitosan is prone to form an intermolecular hydrogen bond in aqueous media due to its hygroscopic nature. One study show that the moisture content of medium molecular weight chitosan from 7% to 11% (w/w) and doesn’t depend on DD or MW of polymer which is in agreement with our study (Costantino, Langer, & Klibanov, 1994; Garr & Rubinstein, 1992; Rege, Shukla, & Block, 1999). In this research, the moisture content of 3.5% after storage for 6 months at 40°C/75% RH indicated that it did not affect stability and instead represented stable hydrogen bonding which were formed during the formulation process.
Inter-complex network formation between Chitosan and PVA
The enhanced stability of the nanoformulation was offered by physical modification of chitosan into PEC. This formed PEC, (by the chemical interaction between positively charged medium molecular weight chitosan and neutrally charged PVA) prevent the ionization of chitosan –NH2 functional group (Mucha, Ludwiczak, & Kawinska, 2005). Here, the Tween 80 and the PVA which not only act as cross linking polymer and also as effective stabilizer are responsible for stability (Hafner, Durrigl, Pepic, & Filipovic-Grcic, 2011; Lubben et al., 2003).
Conclusion
Most of the studies based on either evaluation of drug stability or polymer stability. When preparing polymer encapsulated drug formulation, especially nanoformulation, alteration in both drug and polymer characteristics results. In this case, both drug and polymer synergistically enhance their properties due to increased stability or adversely lower their existing properties because of decreased stability. In such a case, determination of stability becomes important to confirm formulation characteristics for further preclinical or clinical evaluation. In this paper, the various stabilizing effect of chitosan were proved by various stability studies.
Increasing the bonding degree between chitosan and simvastatin apparently stabilizes the nanoformulation. Choice of appropriate humid conditions is vital, for storage of chitosan-based pharmaceuticals. Since no standard references have been provided, several research studies confirmed the rate of hydration of chitosan pharmaceuticals at high RH and high temperature. In our study, we found the stability of nanoformulation in higher humid and temperature condition, which indicate that the nanoformulation can be stored in higher humid/temperature condition. Since the photostability was found to be less, the protection from light should be ensured during storage. Development of this chitosan-based nanoconjugate needs to be proved in vivo to recognize its applications. Therefore improved pharmacokinetics and pharmacodynamics studies of this nanoformulation will be presented in our future paper.