Optimization of linseed cultivation, a promising way to enhance its secoisolariciresinol diglucoside lignan content
Abstract
There is strong evidence on the anticancer properties of secolariciresinol diglucoside (SDG), the predominant flaxseed lignan, especially for mammary and prostatic carcinomas. Although the effect of cultivar and location on flaxseed SDG level has been reported, much less is traced regarding the influence of agronomical treatments. The purpose of this study is to determine if agricultural management practices could enhance SDG accumulation in flaxseed. We studied the effect of irrigation frequencies (I), added nutrients (N, zinc and humic acid) and fertilization treatments (F) and their interactions on SDG level and seed yield of the Egyptian flax cultivar Giza 9. Samples were extracted and analyzed for SDG, after alkaline hydrolysis, using HPLC-UV method. The concentration of SDG and its yield varied between 415.55-845.95 mg/100 g whole seeds and 1.77-4.05 Kg/feddan on a dry weight basis, respectively according to different agricultural treatments. I, N and F factors and their interactions had a significant effect on SDG. I × N × F interaction contributed to the majority of SDG variation (31.81 %), followed by I factor (27.96 %), whereas seed yield was more affected by F factor (72.7 %). The I × F interaction was the only significant interaction on the seed yield contributing only for 0.43% of the variance. Moderate irrigation frequency with the humic acid foliar application and mineral nitrogen fertilizer was the best combination for achieving the highest SDG levels. Using optimum agronomic practice would benefit the nutraceutical industry by optimizing flaxseed lignan content and hence enhancing its anticancer, health-promoting effect.
Keywords
Agronomical treatments, Fertilization, Flaxseed, Humic acid, Irrigation, Lignan, SDG
Introduction
Flax (Linum usitatisimum L.) is an economically important oilseed and fiber crop. The seeds are characterized by nutritional oil rich in α-linolenic acid (omega-3 fatty acid) as well as good quality protein (Bernacchia, Preti, & Vinci, 2014). Flaxseed is considered as the richest source of plant lignans (Thompson, Robb, Serraino, & Cheung, 1991) with secoisolariciresinol diglucoside (SDG) being the predominant one (Hano et al., 2006). SDG has shown to be protective against certain types of cancers (i.e. breast, lung and colon) because of its antioxidant, antiproliferative, anti-estrogenic or anti-angiogenic properties or possibly due to its ability to inhibit certain enzymes (Carreau, Flouriot, Bennetau-pelissero, & Potier, 2008).
In flaxseed, SDG is not found free but rather as an ester-linked with 3-hydroxy-3-methyl glutaric acid (HMGA) and other phenolic compounds, such as p-coumaric and ferulic acids glycosides forming SDG oligomers (Struijs, Vincken, Doeswijk, Voragen, & Gruppen, 2009). Due to the health benefits of SDG, several methods have been used for its quantification including high performance liquid chromatography (HPLC) with photodiode array (PDA) detection (Eliasson, Kamal-Eldin, Andersson, & Aman, 2003; Johnsson, Kamal-Eldin, Lundgren, & Aman, 2000), colorimetric electrode array (CEA) detection system (Kraushofer & Sontag, 2002) and MS/MS detectors (Popova, Hall, & Kubatova, 2009) or GC/MS (Thompson, Boucher, Liu, Cotterchio, & Kreiger, 2006). Great variation in SDG level has been observed in flaxseed in the reported literature. This variation could be due to different methods of extraction and/or analysis. Moreover, the effect of variety, growing location and harvest year on the SDG content has been reported by many authors (Chen, Liu, Shi, & Ma, 2007; Johnsson et al., 2000; Thompson et al., 2006; Zimmermann, Bauermann, & Spedding, 2007). In this aspect, (Fuentealba, Figuerola, Estévez, Gon-zález-muñoz, & Muñoz, 2015) found that the harvest year did not influence flaxseed cultivars in the same manner. Nevertheless, cultivation of a flaxseed cultivar (Sorrel cultivar) in two different locations had no significant effect on its SDG content, though other tested cultivars were significantly influenced. According to several studies, the content of SDG varied between 9 to 30 mg/g in defatted flaxseed powder and 6 to 13 mg/g in whole flaxseeds on dry weight basis (Chen et al., 2007; Eliasson et al., 2003; Fuentealba et al., 2015; Johnsson et al., 2000).
It has been shown that different irrigation regimes, as well as different fertilization sources, influence secondary metabolites content in different plants (Hassan & Ali, 2014; Mohamed, Wahba, Ibrahim, & Yousef, 2014; Sousa et al., 2008). The effect of different agricultural treatments on some flax yield components, such as the yields of seed, straw, fiber and oil as well as growth parameters has been extensively studied (Bakry, Nofal, Zeidan, & Hozayn, 2015; Mousa, El-Kady, & Zedan, 2010; Rashwan, Mousa, El-Sabagh, & Barutcular, 2016). However, the influence of different agronomic practices on the accumulation of lignans in linseed is scarce. Only two reports have been traced on the effect of nitrogen fertilization on the lignan content of flaxseed cultivars, grown in Canada, Germany and Spain, showing variable results being influenced by cultivar effect to some extent (Muir & Westcott, 2000; Zimmermann et al., 2007). So far, no study has explored other agricultural treatments and their interactive effect on flaxseed lignans. As a new promising approach, optimization of linseed cultivation to enhance flax lignan levels seems necessary. The purpose of this work is to determine if agricultural practices could increase SDG accumulation in flaxseed. In our previous work (Ezzat et al., 2018), Giza 9 was the richest cultivar in SDG, among other investigated Egyptian cultivars, showing the most significant cytotoxic activity against ER-receptor positive human breast cancer cell lines; MCF7 and T47D. Furthermore, we reported that the aforementioned cultivar yielded seed oil with the highest omega-3 polyunsaturated fatty acid content, compared to other investigated Egyptian flax cultivars, and exhibited anti-depressant-like effect in a rat model of postpartum depression, comparable to that of fluoxetine (Tanbouly, 2017). Therefore, this study was conducted to examine the influence of different irrigation treatments, some nutrients (zinc and humic acid) as well as fertilization treatments and their interactions on biomass production and SDG accumulation in flaxseed cultivar, Giza 9, a promising medicinally valuable cultivar.
Materials and Methods
Chemicals
All solvents used were of HPLC grade and were purchased from E. Meck. A reference sample of seco-isolariciresinol diglucoside (SDG) was purchased from Chroma Dex (Santa Ana, CA, USA).
Experimental design
Flaxseed cultivar Giza 9, which represents the most widely cultivated commercial cultivar in Egypt, was grown at Al Gemmiza Agric. Res. Station Farm, Gharbia Governorate A.R.C, Egypt during the winter season of 2013/2014. This farm is located at 30o 44' 50" N, 31o 07' 50" E, with clay soil and an average rainfall of 70 mm. Mechanical and chemical analyses of the experimental soil are listed in Table 1 and were carried out according to (Chapman & Pratt, 1962). The area of each plot was 10.5 m2, in which seeds were sown in rows 3.5 m long with 20 cm row spacing. The sowing date was 19 November 2013. The experimental design was a split-split plot design with three replications. Three irrigation treatments (I), I1 (one irrigation), I2 (two irrigations) and I3 (three irrigations) after sowing irrigation, were assigned to main plots with the first one being applied after 24 days from planting. The irrigation quota was 400 m3 feddan-1 in each time, with River Nile water used for irrigation treatments. All water requirements of the plant were achieved through surface irrigation. Two nutrients (N), zinc (NZn, as Zn SO4) and humic acid (NHm, as Trisol activator), were assigned to subplots at a rate of 100 ppm feddan-1 by foliar spray after 90 days from sowing. The sub-subplots (10.5 m2) were allocated to different fertilization treatments (F), three nitrogen sources and zero nitrogen (F0). Mineral nitrogen, as calcium nitrate (F1), was applied three weeks after planting at a rate of 45 kg feddan-1, as recommended by the Egyptian Ministry of Agriculture. Organic nitrogen, as compost (F2) (0.5% organic N) was applied two weeks before sowing during land preparation at a rate of 250 Kg feddan-1. Biologic nitrogen, as nitrobien (the commercial name of nitrogen-fixing bacteria containing Azotobacter sp. and Azospirillum sp.), was obtained from the General Organization for Agricultural Equalization Fund (GOAEF), Agricultural Research Center, Giza, Egypt. Flaxseeds were washed and immersed in Arabic gum (an adhesive material to render their surface sticky), allowed to dry, then inoculated with the biofertilizer specific bacteria just before planting at a rate of 1 kg feddan-1. All agronomic practices were applied at the proper stage of development. At the maturity stage, seeds were harvested by hand and cleaned of debris prior to laboratory testing.
Values |
Soil characters |
---|---|
Mechanical analysis |
|
Organic matter (%) |
1.7 |
Coarse sand (%) |
6.63 |
Fine sand (%) |
16.32 |
Silt (%) |
31.92 |
Clay (%) |
45.13 |
Texture |
Clay loam |
Chemical analysis |
|
pH |
7.98 |
N (ppm) |
26.61 |
P (ppm) |
15.88 |
K (ppm) |
315.15 |
EC (ds m-1) |
1.16 |
CaCO3 (%) |
2.56 |
Standard Preparation
The standard stock solution was prepared by dissolving 20 mg of SDG in 10 ml methanol, with a final concentration of 2 mg/ml. Serial dilutions of SDG standard solutions were prepared in the range of 125-2000 µg/ml. The regression equation was: y = 7.3977 - 387.17 [R2 = 0.9975].
Sample preparation
Each sample of flaxseed (50 g) was milled using a coffee grinder, followed by defatting with n-hexane (3×400 ml) at 60oC using an ultrasonic bath for 30 min. The defatted powder was then extracted with 80% v/v aqueous methanol at 60oC for 1 hr (3×400 ml) (Popova et al., 2009). The resulting methanolic extract was filtered, pooled and concentrated under vacuum (at 60oC) till complete dryness. The methanolic extract was subjected to alkaline hydrolysis adopting the method of (Muir & Westcott, 2000). The extract (about 100 mg), accurately weighed into a 25 ml volumetric flask, was hydrolyzed using 2.0 ml of NaOH (0.5N) for 3 hr at room temperature. The hydrolysate was then neutralized with acetic acid (0.5N) and completed with methanol to 25 ml, filtered through 0.45 µm membrane filter and subjected to HPLC analysis. All samples were analyzed in triplicates.
HPLC analysis
HPLC analysis was carried out, as previously described (Ezzat et al., 2018), using Agilent 1100 series HPLC system (Agilent Technologies, Palo Alto, CA) equipped with a quaternary pump G1311A, degasser G1322A, UV detector and Agilent Chem Stations software. The mobile phase consisted of acetonitrile (solvent A) and 0.3% phosphoric acid (solvent B) using a gradient elution program, as follows: 11% to 25% A/B in 15 min, 25% to 60% A/B in 10 min, 60% to 80% A/B in 5 min and 80% to 11% A/B in 5 min. The separation was carried out on a LiChrospher® RP-18 HPLC column (250x4.6 mm, 5 µm; Merck, Germany). The flow rate was 0.9 mL min-1, and the injection volume was 20 µl. The analysis was carried out at room temperature, and the detection wavelength was set at 280 nm.
Statistical analysis
SPSS (SPSS for Windows, Version Rel. 10.0.5., 1999, SPSS Inc., Chicago, IL) was used for statistical analysis. Factorial design analysis of variance (ANOVA) was performed using a general linear model (GLM), with irrigation, plant nutrients and fertilization treatments as fixed effects. There were 3 levels for irrigation, two levels for nutrients and four levels for fertilization treatments, with three replicates for each sample. Tukey’s HSD post hoc test was performed to compare differences between means. Correlation analysis was performed using a two-tailed Pearson's correlation test. Statistical significance was declared at p < 0.05 (a > 0.95), p < 0.01 (a > 0.99). For field data, a univariate procedure (GLM) was employed to assign the necessary error terms to the main plot, sub-plot and sub-subplot factors.
Results and Discussion
Analysis of variance of agricultural treatments (experimental factors)
The significant variation in the seed yield of Giza 9 cultivar was mainly due to the individual effects of F, and I factors, being more affected by F (72.7%) than I (25.7%) (p˂0.01, Table 2). This is in contrast to the finding of (Gabiana, Mckenzie, & Hill, 2005) who reported a great variation in flaxseed cultivar Hinu in response to irrigation and little response to nitrogen. However, other factors such as soil nitrogen level, soil moisture or season may contribute in this variable response to nitrogen (Matheson, 1976). On the other hand, the N factor showed a very small influence on seed yield (0.52%) (p˂0.01,Table 2). Nonetheless, only one significant interactive effect (IxF) was shown, yet it revealed the least influence on seed yield accounting only for 0.43% of the variance. Other interactions (I×N×F, I×N and N×F) revealed no significant effect on the seed yield.
Source of variation |
DF |
Seed yield a |
Seed yield b |
SDG a |
SDG b |
---|---|---|---|---|---|
I |
2 |
35550.50** |
25.71 |
153326** |
27.96 |
N |
1 |
722.00** |
0.52 |
17385** |
3.17 |
F |
3 |
100514.90** |
72.70 |
71642** |
13.06 |
I × N |
2 |
38.60 ns |
0.03 |
38485** |
7.02 |
I × F |
6 |
588.50** |
0.43 |
50274** |
9.17 |
N× F |
3 |
40.80 ns |
0.03 |
41889** |
7.64 |
I × N × F |
6 |
146.00 ns |
0.11 |
174471** |
31.81 |
Error |
48 |
654.70 |
0.47 |
944 |
0.17 |
Results are expressedas a sum of squares and b percent of sum of squares; **p˂ 0.01; DF: degrees of freedom; ns: not significant.
There were significant effects of I, F and N individually as well as their interactions on SDG content in Giza 9 cultivar (p˂0.01,Table 2). However, the effect of I×N×F interaction accounted for the majority of variance, followed by the effect of I (31.81, 27.96 %, respectively, p ˂ 0.01,Table 2). F was responsible for higher variation in SDG content than the interactive factors: I×F, N×F and I×N, ranging from 7.02 to 13.06 %. There was a relatively very small effect of N factor on the variation of SDG content in the current study contributing only to 3.17% of the variance (p ˂ 0.01, Table 2). These results indicate the possibility of enhancing SDG content in flaxseed crop through optimizing the combination of integrative agronomic treatments. Albeit, selecting optimum irrigation frequency should come in the first step, followed by the appropriate use of nitrogen fertilization source.
Effect of irrigation
Effect of irrigation on seed yield
From Table 3, different irrigation treatments significantly affected flaxseed yield of Giza 9, being increased by increasing irrigation times. The highest values were recorded under I3 (477.4Kg/feddan), followed by I2 (453.2Kg/feddan) revealing 12.9 and 7.2 % increase, respectively when compared to I1 (422.9 Kg/feddan). These results are in agreement with those reported by (Chorumale, Dahatonde, & Vyas, 2001) (Yenpreddiwar, Nikam, Thakre, Harsha, & Sharma, 2007) who found that two irrigations applied at flowering and capsule-filling stages significantly increased flaxseed yield, in India, compared with no irrigation or one irrigation only at the flowering stage. Linseed was the first crop to be affected by water stress, among other studied crops, due to its shallow root system (Hocking, Kirkegaard, Angus, Gibson, & Koetz, 1997). This can also be ascribed to the vital role of water supply at adequate amount on different plant physiological processes, such as photosynthesis, transpiration, respiration and enzymatic reactions resulting in better growth of crop plants and consequently higher yield production (Hassan & Ali, 2014; Hwary & Yagoub, 2011). In accordance, a significant increase in flaxseed yield was observed under adequate water supply (Gabiana et al., 2005; Rashwan et al., 2016). However, a controversial report showed that irrigation had no significant impact on flaxseed yield (Bauer, Stone, Foulk, & Dodd, 2015).
Irrigation (I) |
Seed yield |
SDG content |
SDG yield* |
|||
---|---|---|---|---|---|---|
Mean (Kg/feddan) |
SD |
Mean (mg/100 g seed) |
SD |
Mean (Kg/feddan) |
SD |
|
I1 (one time) |
422.9 c |
39.97 |
520.7 c |
70.70 |
2.21 c |
0.4 |
I2 (two times) |
453.2 b |
38.24 |
622.3 a |
108.80 |
2.83 a |
0.63 |
I3 (three times) |
477.4 a |
37.49 |
528.52 b |
27.27 |
2.53 b |
0.28 |
SDG yield, calculatedas the product of SDG content and seed yield; Means within the same column,followed by different letters are statistically different at P ˂ 0.05,according to Tukey's HSD post hoc test.
Effect of irrigation on SDG content and yield
A significant difference in SDG content was observed among different irrigation treatments in our study (Table 3). This is in agreement with some reports where different water managements have shown to affect the levels of plant secondary metabolites, such as phenolics (Helyes, Lugasi, & Pek, 2012; Khosh-Khui, Ashiri, & Saharkhiz, 2012). In the present study, the highest amount of SDG (622.3mg/100 g) was accumulated in flaxseeds subjected to two irrigations, 17.7% higher compared to plants under I3 (Table 3).
These results are not consistent with the findings of (Romero, Tovar, Girona, & Motilva, 2002) who has studied the effect of irrigation regime on olive oil lignans. Under different deficit irrigation strategies, lignans increased in olive oils from the most irrigated treatments. Albeit, later on, (Caruso et al., 2014) observed no change in the concentrations of lignans, (+)-1-acetoxipinoresinol and (+)-1-pinoresinol in olive oil, being unaffected by the irrigation regime in their report. The reason of this conflict may be due to that different plants might respond differently to different water practices. Nevertheless, the use of different water regimes, such as irrigation scheme, frequency or volume, in addition to other environmental factors, may lead to different results in different trials. It should also be taken into consideration that different biotic and abiotic stresses, such as infection, pathogen attack, cold and high-temperature stresses as well as nutritional stress, notably nitrogen, phosphate or iron limitations have a great influence on the levels of phenylpropanoids in different plants (Dixon & Paiva, 1995). This may explain why different cultivars and locations show variable contents of the same secondary metabolites (Thompson, Rickard, Cheung, Kenaschuk, & Obermeyer, 1997) (Piispanen, Willför, Saranpää, & Holmbom, 2008). In our study, moderate irrigation frequency resulted in the highest amount of SDG (622.3 mg/100 g) in flaxseeds compared to higher irrigation frequency. This might be attributed to the increase in the activity of phenylalanine ammonia-lyase (PAL) resulting in an increase in total phenolics production under low soil field water capacity (Jaafar, Ibrahim, & Fakri, 2012).
Similarly, the SDG lignan yield reached its maximum (2.83 Kg/feddan) under I2, followed by I3 (2.53 Kg/ feddan), with the lowest yield under I1 (Table 3). Hence, in our study, different irrigation treatments have shown much more influence on SDG concentration compared to seed yield. Optimum selection of irrigation practice will result in the production of higher flaxseed lignan in Giza 9 cultivar.
Effect of added nutrients
Effect of added nutrients on seed yield
Added nutrients showed a significant effect on flax seed yield (0.52%), with the least contribution compared to other agronomical treatments (p ˂ 0.01,Table 2). Zinc application showed a slightly higher significant increase (1.4%) than that of humic acid (Table 4). These results are in agreement with those reported by (Bakry, Ibrahim, Eid, & Badr, 2014; Bakry et al., 2015; Bakry, Sadak, El-Karaman, & F, 2015) recording a higher seed yield, under newly reclaimed sandy soil in Egypt, in response to foliar sprays of humic acid or zinc compared to control plants. The increase in seed yield in response to zinc may be attributed to its necessity for auxin synthesis, in particular, indole acetic acid, which acts as growth promoting substance (Alloway, 2008; Marschner, 1995). In addition, zinc has a key role as a structural, functional or regulatory cofactor of various enzymes involved in different biochemical pathways, together with its importance for protein synthesis due to its involvement in genetic material function and stability (Alloway, 2008; Marschner, 1995) and consequently plant growth and yield. Nevertheless, the stimulatory effect of humic acid on flaxseed yield can be ascribed to its hormone-like activity (Nardi, Pizzeghello, Muscolo, & Vianello, 2002; Zandonadi, Canellas, & Facanha, 2007) and enhanced nutrient absorption through increased cell permeability (Valdrighi et al., 1996). Moreover, humic acid has shown to improve soil fertility and structure (Bronick & Lal, 2005) in addition to enhancing soil microbial population (Valdrighi et al., 1996), hence leading to higher nutrients available for plants (Trevisan, Francioso, Quaggiotti, & Nardi, 2010).
Effect of added nutrients on SDG content and yield
SDG content was significantly affected by tested plant nutrients. The humic acid foliar application resulted in 5.7% more lignan (572.7 mg/100 g) compared to that of zinc (541.6 mg/100 g) (Table 4). This increase may be attributed to the stimulatory effect of high molecular weight humic substances on the phenylpropanoid metabolism. This could be confirmed by the induction of phenylalanine-ammonia-lyase (PAL) and tyrosine-ammonia-lyase (TAL) enzyme activities and increased accumulation of phenolic compounds (phenolic acids and flavonoids) (Schiavon et al., 2010). Furthermore, humic substances contain a cytokinin in physiologically active concentrations, which can stimulate plant metabolism (Pizzeghello, Francioso, Ertani, Muscolo, & Nardi, 2013). Moreover, (Bakry et al., 2014; Bakry et al., 2015; Bakry et al., 2015) reported that humic acid application caused significant increases in phenolic contents in the flax plant, grown under newly reclaimed sandy soil in Egypt, compared to control plants, being in agreement with our results. However, (Krajčova, Schulzova, Hajšlova, & Bjelkova, 2009) reported that neither different humic acid sources (Trisol Fructus and Trisol activator) nor different amounts of zinc added in fertilizers had a significant effect on the lignan content of flax cultivars. The SDG yield was affected in the same way showing higher yields (2.57 Kg/feddan) under humic acid application, compared to that of zinc (2.47 Kg/feddan) (Table 4). Thus, added nutrients resulted in more variation in SDG content than in seed yield.
Nutrient (N) |
Seed yield |
SDG content |
SDG yield* |
|||
---|---|---|---|---|---|---|
Mean (Kg/feddan) |
SD |
Mean (mg/100 g seed) |
SD |
Mean (Kg/feddan) |
SD |
|
NZn (Zinc) |
454.3 a |
44.11 |
541.6 b |
55.00 |
2.47 b |
0.42 |
NHm (humic acid) |
448.0 b |
44.54 |
572.7 a |
111.30 |
2.57 a |
0.6 |
*SDG yield,calculated as the product of SDG content and seed yield; Means within the same column, followed by different letters are statistically different at P ˂ 0.05,according to Tukey's HSD post hoc test
Effect of fertilization
Effect of fertilization on seed yield
From the results in Table 5, different fertilization treatments showed a significant effect on flaxseed yield. Throughout the literature, the effect of nitrogen on seed yield was conflicting showing favorable increase (Mousa et al., 2010), decrease (Zimmermann et al., 2007) or no effect (Woodhead & Neilson, 1976). In the current study, different nitrogen fertilization sources revealed a significant increase in seed yield from 20.9 to 23.6% compared to unfertilized plants (Table 5), being consistent with the results reported by (El-Nagdy, Nassar, El-kady, & Yamanee, 2010) (Mousa et al., 2010). These results can be attributed to the small amount of nitrogen available to plants in soil. Thus, the use of fertilizers is important for increasing agricultural crops yield through increasing the availability of nitrogen supplies to plants (Gendy, Ahl, & Mahmoud, 2012). The highest estimates of seed yield were achieved by applying mineral fertilizer (478.1 Kg/feddan), followed by organic and bio-fertilizers showing a slight but significant decrease of 1.3 and 2.2 %, respectively when compared with mineral one, which is in agreement with the finding of (El-Nagdy et al., 2010). Nevertheless, (Salem, Zidan, & Esmail, 2006) indicated the response of flax plants to biofertilizers and recorded maximum values of yield and quality.
Fertilization |
Seed yield |
SDG content |
SDG yield* |
|||
---|---|---|---|---|---|---|
treatment (F) |
Mean (Kg/feddan) |
Mean (Kg/feddan) |
Mean (mg/100 g seed) |
SD |
Mean (Kg/feddan) |
Mean (Kg/feddan) |
F0 (No fertilizer) |
386.8 d |
25.62 |
516.8 d |
47.30 |
2.00 d |
0.23 |
F1 (Mineral fertilizer ) |
478.1 a |
26.26 |
604.3 a |
124.00 |
2.89 a |
0.58 |
F2 (Organic fertilizer) |
472.0 b |
19.56 |
546.4 c |
59.60 |
2.58 c |
0.32 |
F3 (Biofertilizer) |
467.7 c |
22.16 |
561.1 b |
88.00 |
2.63 b |
0.43 |
*SDG yield,calculated as the product of SDG content and seed yield; Means within the samecolumn, followed by different letters are statistically different at P ˂ 0.05,according to Tukey's HSD post hoc test
Effect of fertilization on SDG content and yield
The results in Table 5 showed that SDG concentration responded to different fertilization treatments. In this study, the use of three different nitrogen sources significantly enhanced the SDG content in the Egyptian flaxseed cultivar (Giza 9) from 5.73% under F2 to 16.93% under F1, when compared to plants receiving no nitrogen supply (Table 5). Our results do not agree with (Piispanen et al., 2008) (Zimmermann et al., 2007) who reported that nitrogen fertilization had no significant effect on the concentration of lignans in Norway spruce and flaxseed cultivars, respectively. Though, our results partly match those of (Westcott, Muir, Lafond, & Mcandrew, 2002), who reported (Song et al., 2015) (Westcott et al., 2002) a different response to nitrogen supply between high and low SDG flaxseed cultivars, grown in Canada. Nitrogen boosted flax yield but resulted in reductions in SDG in the higher SDG flaxseed cultivars (AC Emerson and AC McDuff cultivars), albeit the Flanders cultivar (low SDG cultivar) showed a modest increase in SDG as nitrogen rate increased, being the only cultivar-nitrogen interaction with statistical significance at p < 0.01. The higher SDG contents, detected under different fertilization treatments, may be cultivar related or could be attributed to the sufficient availability of nitrogen from these treatments, which in turn resulted in more phenylalanine amino acid for the formation of polyphenols after its utilization in protein biosynthesis and release from protein molecules during their turnover (Margna, 1977). This is further evidenced by the increased growth parameters (plant height and technical length) of flax plant in our study (data not shown).
Regarding the SDG lignan yield, the same pattern was noticed with the highest yield observed on applying mineral fertilizer (2.89 Kg/feddan) and the lowest one observed under no fertilization treatment (2.0 Kg/feddan) (Table 5). This shows that different fertilization treatments had more influence on SDG content than biomass yield.
Although nitrogen is one of the most important nutrients for crop production and influence secondary plant metabolites content and hence their quality, its interactions with other nutrients as well as the combination with various environmental conditions as light intensity, plant species, genotype and location are complex factors affecting the accumulation of secondary metabolites in plants (Hassan, 2012). Therefore, our results suggest the feasibility of achieving high lignan yield and better flax quality, under the current environmental factors, by using the optimum fertilization treatment.
Interactive effects of irrigation, fertilization and nutrients on seed yield and SDG content
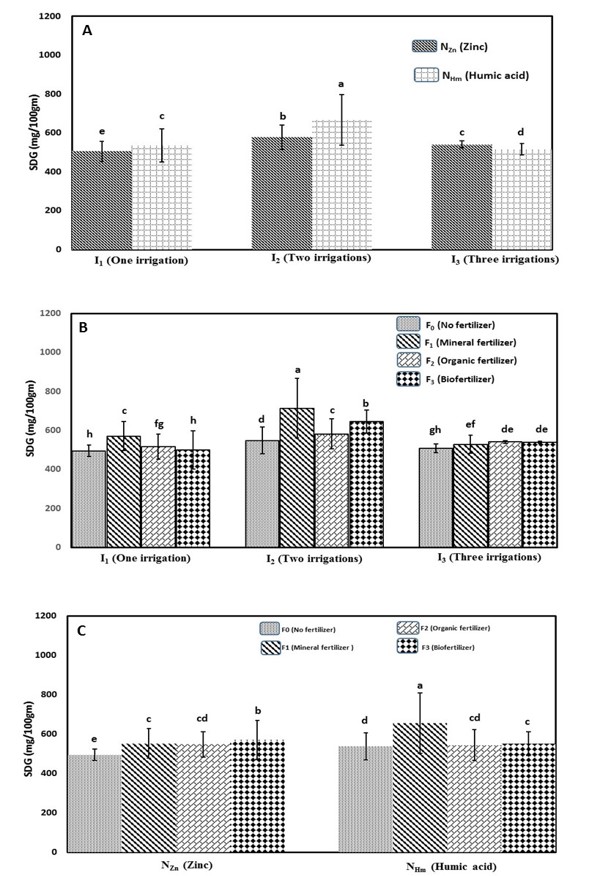
Significant interactive effects of the different applied agronomical treatments on SDG content have been shown in this study (p ˂ 0.01, Table 2). However, for the seed yield, only one interactive effect (IxF) was significant. Further analysis of significant interactive effects is shown in Figure 1, Table 6,Table 7, and is discussed below.
Agricultural treatment |
Seed yield* |
||
---|---|---|---|
I1 (One irrigation) |
I2 (Two irrigations) |
I3 (Three irrigations) |
|
F0 (No fertilizer) |
357 ± 4.07 i |
388 ± 4.93 h |
415.5 ± 7.74 g |
F1 (Mineral fertilizer ) |
444.9 ± 5.55 ef |
482.5 ± 5.58 c |
507 ± 4.4 a |
F2 (Organic fertilizer) |
449.4 ± 2.04 e |
472.5 ± 6.48 d |
494 ± 6.92 b |
F3 (Biofertilizer) |
440.5 ± 2.94 f |
469.6 ± 4.5 d |
493 ± 3.93 b |
Seed yield expressedas M±SD, Kg/feddan; Different letters represent significant difference at P ˂0.05, according to Tukey's HSD post hoc test
For seed yield, IxF interaction was the only significant interactive effect in this study, though its contribution was very small accounting only for 0.43 % of the variance (p ˂ 0.01, Table 2). As shown in Table 6, the highest seed yield was recorded under I3 F1, followed by I3 F2 and I3 F3 (507±4.4, 494±6.92 and 493±3.93 Kg/feddan, respectively), whereas lowest seed yields were observed under I1 F0 and I2 F0 (357±4.07 and 388±4.93 Kg/feddan, respectively).
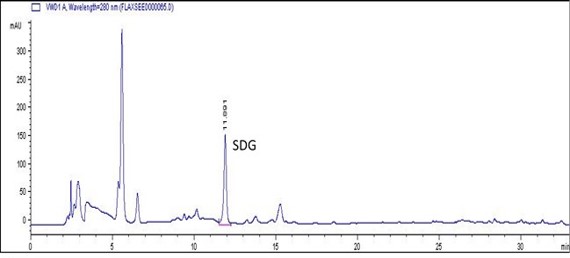
Agricultural treatment |
SDG content (mg/100 g seed)* |
|||||
---|---|---|---|---|---|---|
I1 (One irrigation) |
I2 (Two irrigations) |
I3 (Three irrigations) |
||||
NZn (zinc) |
NHm (humicacid) |
NZn (zinc) |
NHm (humicacid) |
NZn(zinc) |
NHm (humicacid) |
|
F0 (No fertilizer) |
470.29 ± 2.88 mn |
519.40 ± 3.62 i-k |
488.07 ± 5.66 lm |
607.84 ± 5.27 d |
527.53 ± 1.29 h-j |
487.61 ± 4.24 lm |
F1 (Mineral fertilizer ) |
505.90 ± 12.90 kl |
635.94 ± 5.06c |
580.89 ± 10.95 ef |
845.95 ± 0.94 a |
569.12 ± 1.73 fg |
487.77 ± 5.01 lm |
F2 (Organic fertilizer) |
461.86 ± 6.12 n |
572.20 ± 5.80 ef |
649.08 ± 3.79 c |
514.63 ± 4.56 jk |
534.29 ± 0.69 h-j |
546.37 ± 0.54 gh |
F3 (Bio fertilizer) |
584.20 ± 15.90 d-f |
415.55 ± 5.69 o |
594.68 ± 8.97 d-f |
696.85 ± 3.46 b |
533.38 ± 0.10 h-k |
542.12 ± 4.39 hi |
*SDG content expressedas M±SD, mg/100 g on a dry weight basis of seed; Different letters represent significant difference at P ˂ 0.05, according to Tukey's HSD post hoc test
As shown in Figure 1, the (IxN) and (IxF) interactions had significant influence on the SDG content showing the highest concentrations under I2 NHm and I2 Nzn (666.3, 578.2 mg/100 g, respectively) as well as under I2 F1, I2 F3 and I2 F2 treatments (713.4, 645.8 and 581.9 mg/100 g, respectively). The lowest levels of SDG were observed under I1 F0, I1 F3 and I3 F0 treatments (494.8, 499.9 and 507.6 mg/100 g, respectively) as well as under I1 Nzn and I3 NHm treatments (505.6 and 516mg/100 g, respectively). This revealed that all flaxseed samples under I2 (subjected to two irrigations) contained the highest SDG among other samples, regardless of the use of different added nutrients or fertilizers. Furthermore, the lowest values of SDG were noticed under I1 and I3, regardless of other agronomical treatments. These findings can further support that irrigation accounts for the largest variation in SDG content (27.96%, p ˂ 0.01, Table 2). It is well known that suitable irrigation can increase the soil water content and also the nutrient availability, and as a result increase the crop uptake and efficiency of fertilizer (Rego, Grundon, Asher, & Edwards, 1988). On the other hand, higher irrigation levels may cause nitrogen leaching, thus reducing the availability of soil nitrogen to plants (Niaz et al., 2004) (Tarkalson, Payero, Ensley, & Shapiro, 2006). Thus, it can be observed in this study that optimum irrigation frequency is a prerequisite for different fertilizers or added nutrients to enhance nitrogen absorption. SDG content was significantly increased at low irrigation frequencies (under I1 and I2) by the application of mineral fertilizer, compared to other fertilization treatments. This can be attributed to the availability of more nitrogen in soil on applying inorganic fertilizer, which improves water use efficiency in plants and consequently minimizes the detrimental effects of water stress (Waraich, Ahmad, Ashraf, Saifullah, & Ahmad, 2011). Thus, adequate nitrogen fertilization can mitigate the negative effect of water stress.
The effect of NxF interaction was significant on flax SDG, with the highest concentrations under F1 NHm and F3Nzn treatments (656.6 and 570.8 mg/100 g, respectively), while the lowest values under F0 Nzn treatment (495.3 mg/100 g). Thus, the added nutrients effect on SDG depended mainly on either irrigation or fertilization treatments supporting their little contribution (3.17%, p ˂ 0.01, Table 2) to SDG variation under this trial. This can also be ascribed to that both humic acids and zinc applications can significantly increase phenolic compounds (Marichali et al., 2016) (Schiavon et al., 2010) (Song et al., 2015), but their interactions with other agronomical treatments may result in variations in phenolic contents.
IxNxF interaction had a statistically significant effect on the SDG level of flaxseed, accounting for the majority of variance in this study (31.81 %, p ˂ 0.01, Table 2). As shown in Table 7, the most enhanced levels of SDG accumulated in plants under I2 F1 NHm (Figure 2), followed by I2 F3NHm treatments (845.95±0.94 and 696.85±3.46 mg/100g, respectively). A similar tendency was observed for SDG yield (4.05±0.05, 3.25±0.05 Kg/feddan, respectively, under the previous treatments, data not shown). On the other hand, lowest SDG levels were recorded under I1 F3NHm, I1 F2NZn and I1 F0 NZn treatments (415.55±5.69, 461.86±6.12 and 470.29±2.88 mg/100g, respectively, Table 7). This further confirmed the greatest contribution of irrigation to SDG variation. Combined use of adequate water supply, appropriate fertilization source and added plant nutrient would enhance flax SDG content.
Correlation between biomass (seed) yield and SDG content
In order to answer whether the higher SDG content in flaxseed, under different agricultural treatments, is due to the higher accumulation of this metabolite or as a result of an expected increase caused by a decrease in plant biomass, the correlation between SDG and seed yield has been studied. A negative correlation has been observed in many reports between biomass yield and other secondary metabolites (Simon, Reiss-Bubenheim, Joly, & Charles, 1992). However, (Zimmermann et al., 2007) revealed nocorrelation between flaxseed yield and SECO or MATA lignans in their report. In the current study, a weak positive correlation was detected between the seed yield and SDG content (r = 0.250, p = 0.035, n=72). Accordingly, enhanced SDG content is not a normal consequence of the decrease in seed yield, but rather due to the higher accumulation of this metabolite. This may indicate that different agronomical treatments tried in this study affected lignan biosynthesis in a certain way.
Conclusion
In conclusion, the studied agronomical factors (I, N and F), as well as their interactions, revealed more impact on SDG content than that on seed yield, in flaxseed cv. Giza 9, except for the F effect. The I×N×F interaction, as well as the irrigation effect, contributed to the highest proportion of variance on SDG content, while seed yield was more affected by fertilization treatments. The concentration of SDG, in the investigated flax cultivar, varied between 415.55 and 845.95 mg/100 g of whole seeds on dry weight basis according to different investigated agricultural treatments. Under the given experimental conditions, application of moderate irrigation frequency (I2) in combination with mineral fertilizer (F2) and humic acid (NHm) was the best combination for achieving maximum concentration of SDG and its yield. The application of bio fertilizer (nitrobien), under the same conditions (I2, NHm), also showed significant pronounced effect on flax SDG content and its yield compared to other treatments. Although many studies have reported the significant effect of cultivar and location on flax SDG, our study revealed that optimum agronomic practice is a promising way that would benefit the nutraceutical industry to optimize flaxseed lignan content and hence enhance its anticancer health-promoting effect.
Conflicts of Interest
The authors declare no conflict of interest.