Recent updates on dry powder for inhalation for pulmonary drug delivery systems
Abstract
Pulmonary drug delivery system is an attractive and most promising approach which is gaining great impetus for the delivery of various therapeutic agents which includes administration of proteins and peptides, agents for the treatment of tuberculosis, asthma, diabetes chronic obstructive pulmonary diseases (COPD), various fungal infections, cancer, etc. This route has gained great importance due to its large surface and the absorptive area, which can potentially bypass the hepatic first-pass metabolism. The dawn of nanotechnology has brought a lot of advancement in the pre-existing formulation aspect with respect to reducing the systemic toxicity and dosing, specific targeting and enhanced efficiency of the treatment. This review reflects on the basics of pulmonary drug delivery, various formulations options available for administration via dry powder inhalers and advances in the same.
Keywords
Pulmonary drug delivery system, deposition and clearance mechanisms, dry powder for inhalation, novel drug delivery systems
Introduction
Pulmonary drug delivery system is an attractive alternative route that is being widely explored for the delivery of various drugs for its respective therapeutic effect. This route has been potentially used for the treatment of the local and systemic diseases like asthma, chronic obstructive pulmonary disease (COPD), tuberculosis, and diabetes, various bacterial and fungal infections, cystic fibrosis, cancer, etc. (Chandel, 2019). The pulmonary route has been exploited since ancient times, i.e. BC 2000 for the treatment of respiratory diseases (Boer, 2017). The ancient therapy included smoking of various substances obtained from various plant origins like leaves or inhalation of vapours from aromatic plants, balsams, etc. In 1764, scientist Philip Stern acknowledged that the only possible way for delivering the medicaments to the lungs was via the windpipe (Sanders, 2007). This finding mainly led to the discovery of the ceramic inhalers used to inhale various therapeutic infusions. An English physician John Mudge coined the word ‘Inhaler' for the first time in 1778. In near 1900s and 20th century, hand-bulb nebulizers and electric & ultrasonic nebulizers were developed respectively. Introduction of developed metered-dose inhalers with propellants was done in 1956, which had a new concept of wet aerosolisation. This device got rapidly popularised owing to its portability, easy usage, inexpensive, fast and salient in comparison to nebulizer equipment. Even though it had many advantages, the patients found difficulty in operating it as compared to nebulizers. In January 1989, the Montreal protocol stated to phase out the production of the substances like chloro-fluoro carbon propellants that were contributing towards depletion of the ozone layer in the stratosphere. This situation gave rise to the development of dry powder for inhalation and dry powder inhalers (DPIs) (Boer, 2017). Dry powder inhalers are being widely used in recent times due to their various benefits like eco-friendly, compact and portable, superior patient adherence and high therapeutic effect. Various concepts of particle engineering and advancement in the inhaler design have also made the drug delivery through DPIs very popular for its usage. This review will mainly present the fate of the particle upon inhalation and recent updates of the various formulation aspects with respect to DPIs.
Materials and Methods
Respiratory Tract and Particle Deposition
The unique physiology of the lungs makes it a potential alternative for drug administration for systemic and local effect (Darquenne, 2006). It possesses great advantages due to its highly vascularized and branched structure, large surface area (>100m2) and nearly 300 million alveoli with a thin alveolar epithelium of 0.1μm. In addition, there is less proteolytic activity compared to the oral route (Darquenne, 2006). These conditions make it favourable for easy mass transfer (Hofmann, 2011).
In addition to the above, the other major advantages over other routes include (Williams, Carvalho, & Peters, 2011).
Needle and pain-free system The quick onset of action Self- medication and patient compliance Reduced repeatability of dose administration Degradation of the active drug via the hepatic pathway is avoided Reduced systemic toxicity Inhalation therapy avoids GI interaction or issues related to poor solubility, reduced bioavailability, gut irritation, drug-food interaction, etc. The prolonged or sustained-release effect can be achieved
The limitations/ drawbacks of the system include (Carvalho & Peters, 2011; Hussain, 2011).
Toxicity and irritation of the drug at the site Instability of the drug in-vivo/in-vitro Clearance mechanisms in the lungs, which include mucociliary and alveolar macrophage clearance. Deposition pattern which includes Particle characteristics like particle size, shape, density, etc. Airway geometry like disease condition, gender, age Breathing patterns like inspiration rate, breath-holding time and tidal volume.
The human respiratory tract is a very complex system and is divided into 3 main parts they are as follows (Hofmann, 2011).
Extrathoracic Region (ET)
Tracheo-Bronchial Region (TB)
Pulmonary Region (P)
The airborne particles that are inhaled via DPIs may be transported to the system via the mouth. Inhalability may be defined as the ability of the particle to enter the head airway based on its aerodynamic diameter. Inhalability has been found to be near 100% for particles smaller than 5μm. The particle size along with the factors mentioned in Figure 1 is very essential for the deep lung penetration, and absorption of the drug or particles from the formulation administrated via DPIs to show systemic effect (Darquenne, 2006). This size is required due to the morphological size of the alveolar sacs with an increase in size, inhalability decreases. Upon inhalation of the medicament, the airflow undergoes varied changes which may lead to various mechanisms of particle deposition. The particle deposition is based on its aerodynamic particle size, momentum, air velocity, the anatomy of the airway path, etc. in different regions of the respiratory tract (Hofmann, 2011).
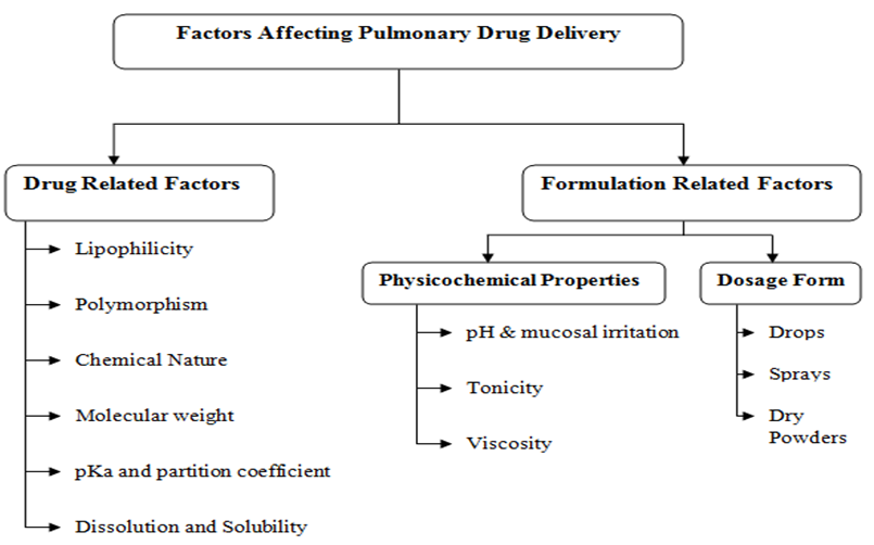
The probable drug deposition mechanisms upon inhalation via DPI are discussed below,
Results and Discussion
Inertial Impaction
This mechanism basically occurs when the particles tend to impact on the walls of the lung airways. This takes place when a particle having large impetus has to change its pathway due to bifurcation of the lungs resulting in impaction of the particle on the wall of the lung airway at the point of deflection. Deposition occurs by particles larger than 5μm via this mechanism. There is a direct proportionality relation between the chance of impaction and size of the particle, air pace and particle density. The sites of particle deposition by inertial impaction include the upper respiratory tract/ upper airways like nose, mouth and pharynx (Darquenne, 2006; Hussain, 2011).
Gravitational Sedimentation
Gravitational force governs this mechanism. The particle deposition occurs at a point when the opposing forces of air equals to the extreme settling speed of the particle. For the above mechanism, there occurs a direct relation between the size and density of the particle; and an inverse relation with the airflow in the lungs. As the airflow decelerates in the regions of bronchiolar and alveolar-interstitial; this mechanism is observed, particularly for particles ranging in the size of 0.5μm - 5μm (Carvalho & Peters, 2011; Darquenne, 2006; Williams et al., 2011).
Brownian Diffusion
In Brownian diffusion, the occurrence of collision is seen between the particles and gas molecules. This cause Brownian motion in which the particles are left suspended and follow a randomised movement for a prolonged period of time. This deposition occurs in areas like bronchioles or alveolar region where the airflow is minimal or nearly absent. Particles possessing aerodynamic size less than or equal to 0.5μm undergo such mechanism. The mechanism is inversely proportional to the particle size (Darquenne, 2006; Hussain, 2011).
Interception
This is mostly an uncommon mechanism unless the particles posses elongated shapes like fibres where the length to diameter ratio is large. The deposition may occur when both the ends of the particle come in contact with the walls of the airway (Darquenne, 2006).
Electrostatic Precipitation
Electrostatic precipitation occurs when the inhaled particles contain some charge on them. The deposition occurs due to the electrostatic attraction between the charged walls of the airways and the charged particles inhaled. This mechanism is not very common either (Darquenne, 2006).
Clearance of Deposited Particles in the lungs
Clearance is the process of eliminating the deposited particle either in the form of transport mechanisms via absorption through blood or the clearance by macrophages (Hussain, 2011). The major clearance mechanisms dominating in the respiratory tract are mucociliary clearance and alveolar macrophages in the upper and lower respiratory airways, respectively (Bo, 1976).
Mucociliary Clearance
This mechanism dominates in the upper respiratory tract, where primary clearance takes place in the nasopharynx and tracheobronchial regions. The mentioned region consists of ciliated columnar and goblet cells. The mucus produced by the goblet cells serves as a medium for entrapment of particles; the entrapped particles are then propelled out of the system with the help of the cilia present. This is a continuous process in which the various particles, including insoluble particles, are eliminated as soon as deposition occurs (Bo, 1976; Williams et al., 2011).
Alveolar Clearance
The insoluble particles deposited in the lower respiratory tract below the ciliated airways are eliminated via the slow phagocytosis; which is further transported within the pulmonary macrophages, which is eventually cleared by the GIT. The pulmonary macrophages are actually immune cells present in the alveolar region responsible for primary clearance; which aids in preventing the bacterial and viral infections. They can easily engulf and digest the external particle since they are rich in lysozymes and other enzymes (Bo, 1976).
Absorption into the circulation system
Movement of the soluble substances and material dissociated into bloodstream regardless of the mechanism is called absorption. Absorption into the blood is a two-stage process, a) the dissociation of the particles into material that can be absorbed into the blood (dissolution) and b) the uptake of material dissolved from particles or of material deposited in a soluble form. Each stage can be time-dependent. Absorption mechanism is a material-specific phenomenon. Soluble inhaled particles can slowly absorb into the blood depending on their absorption properties and their history that is, specific activity (radioactive particles), temperature treatment, and surface area. The particle dissolution rate is a major determinant controlling the rate of absorption for the material retained in the respiratory tract. Absorption clearance takes place in the entire of the respiratory tract with different absorption rates in different regions. Materials have been categorized in fast, medium, and slow materials depending upon their specific dissolution rate.
Dry Powder Inhalers (DPIs)
DPIs are devices that deliver the medicament as the dry powdered formulation of the active substance for local and systemic effect via the pulmonary route. These powdered formulations are formulated as loose agglomerates by various methods by combining the active drug, suitable carriers and dispersing agents. The particle size of the formulation should not be more than 5μm for local effect and not more than 2μm for the systemic effect to be achieved. These devices have proved deep lung penetration by the amalgamation of the performance of the device and powdered formulation. DPIs depend on the patient's inspiratory flow rate; thus devices are developed by taking into consideration children, patients with decreased lung function due to age or disease (Islam & Gladki, 2008; Rogliani & Calzetta, 2017).
Advantages
Simple and compact Patient-friendly and easy to use Deep lung penetration is achieved As the formulation is in powdered form; there will be physical and chemical stability. Available as unit dose, multi-dose, multiple unit dose Monitoring of emitted is possible Free of propellant.
Limitations
Dependent on the patient's inspiratory flow rate Device resistance and dose uniformity Expensive and availability worldwide.
Formulation of DPIs
The process of the formulation can be carried on in three steps:
Production of Active Pharmaceutical Ingredient (API). Blending of API with or without a carrier. Assimilation or incorporation of the formulation into the device (Boer, 2017; Islam & Gladki, 2008).
Development of the DPI formulation may be as follows,
Carrier independent system
This system will not include any incorporation of carrier compounds; the drug either as an independent or in encapsulated form is delivered to lungs via a compatible inhaler device having an aerodynamic diameter of less than 5μm.
Carrier dependent System
This system depends on the inclusion of carrier particles. Addition of such carrier particles improve the flowability of drug particles, increase the dispersing property of cohesive particles upon emission, improves the dosing accuracy, minimise dose variations; increase the inhalation efficiency and easy handling during manufacturing. The carrier particles selected should possess characteristics of physical and chemical stability, must be compatible with other active and inactive ingredients, biocompatible and biodegradable. There are various carriers available to name a few common carriers are lactose, mannitol, alpha-lactose monohydrate, glucose, sorbitol, maltitol, etc (Rahimpour & Kouhsoltani, 2013).
Technologies involved in the production of dry powder for inhalation
It is necessary to produce particles which are suitable for deep lung penetration, i.e. within the range of 1-5μm. The widely used techniques are summarized below, and their comparison is provided in Table 1. (Harrington, Prisk, & Darquenne, 2006; Liang, 2015; Liang, 2018).
Milling
Milling is a process that was conventionally used to obtain dry powders for DPI. The conventional milling technique faced a lot of problems related to its particle shape, size, crystallinity and distribution (Carvalho & Peters, 2011). It produced a particle of >10 µm, which were polydisperse in nature and unsuitable for pulmonary delivery. Thus, the pharma industry employs a jet milling technique to convert the crystalline particle into inhalable particles of desired particle size (Pilcer & Amighi, 2010; Shoyele & Cawthorne, 2006).
The different types of jet mills available are as listed as fluid impact mills, opposed jet mills, spiral jet mill, oval chamber jet mill and fluidised bed opposed jet mill (Nakach, 2004). Basic mechanism involves high-intensity comminution, usually in a fluid-energy (air-jet) mill. This intense milling may lead to a change in the physical (amorphous regions might be generated at the surface of fractured crystals) and the chemical nature of the material. In addition, the charge carried by the particles is also very high, which may result in cohesiveness aggregation and agglomeration of the particles. This will cause a hindrance when the medicament is to be delivered via the inhaler (Lin, 2015; Malcolmson & Embleton, 1998). Although there is an advancement in the design of inhalers, this method is not widely preferred for manufacturing of DPI.
Spray Drying
Spray drying is an entrenched, simple and non-tedious one-step process that is scalable and cost-effective. This method has been in use since the 1990s for the production of biopharmaceuticals such as proteins and peptides (Ameri & Maa, 2006; Shoyele & Cawthorne, 2006). The process of production of dry powders from a liquid system involves atomization of the liquid phase via a spray nozzle into fine droplets, which rapidly undergoes evaporation at high temperature. The vacuum is also applied, which leads to the collection of dry powders in the cyclone chamber of the spray drier.
This method is used for API, excipients or other materials having a high melting point and is capable of resisting the high temperature. Spray drying is advantageous with respect to uniform particle size distribution, small particles, i.e. ≤5 µm which is required for the production of dry powders for inhalation. This method is well established and widely used in both the food and pharmaceutical industry (Sosnik & Seremeta, 2015). The limitations of this process include the processing of thermolabile materials and drug substances. But, this drawback can be tackled by controlling the process parameters or addition of certain excipients that provide protection of the heat-sensitive material and improves the stability.
In recent times, particle engineering, in combination with spray drying technology, can be used to produce porous particles that can be highly respirable and also aids in reducing the dosing. Pulmosphere™ technology involves a unique technique of emulsification-spray drying process to produce highly porous dry particles with enhanced flow and dispersion (Geller, Weers, & Heuerding, 2011). Therefore, the spray drying process in combination with particle engineering strategies is gaining popularity in the dry powder production to provide an alternative therapeutic possibility for patients.
Spray-Freeze Drying
Spray-freeze-drying serves as a substitute for spray drying technology for heat-sensitive substances. An example of a product produced by this technique is Afrezza® DPI; insulin produced using Technosphere® technology. This is a two-step process; firstly, the liquid phase is atomised into a freezing medium (liquid nitrogen, argon, hydrofluoroethers) which convert the spray into frozen droplets.
The latter step includes lyophilisation of the frozen droplets to remove the ice via sublimation process; this results in the production of dry powder. This technique has delivered its importance because of its feasibility and commercial scale-up with respect to the conventional freeze-drying process. The integrity of bio-pharmaceuticals and high production yield is the major advantage of this process (Lin, 2015).
Super Critical Fluid Technology
Super Critical Fluid (SCF) technology is a relatively new powder production method presently under development. This technique produces particles of more uniform size distribution, shape, morphology and crystallinity. The particles will posses lesser charge, which enhances the flowability and dispersibility at the time of discharge from the inhaler device.
The three principle SCF processes include
-
The rapid expansion of supercritical solution (RESS), where particles form as the result of rapid expansion of a supercritical fluid (SF) containing a dissolved solute.
-
Gas antisolvent (GAS) recrystallization, which relies on the capacity of an SF to act as an antisolvent and cause precipitation within a liquid solution or droplet.
-
Solution-enhanced dispersion by supercritical fluids (SEDS) which involves rapid dispersion and mixing of the drug solution with the SF, typically in a coaxial spray arrangement, and extraction of the solvent into the SF, leading to high supersaturation ratios.
Even though it has reported multiple benefits, this technique is still yet to gain impetus in the marketed DPI formulation.
Production Technology |
Advantage |
Disadvantage |
---|---|---|
Jet milling |
Accepted process |
Products formed have poor flowability and solubility with a high surface charge. Instability occurs both physically and chemically due to induced charges |
Spray drying |
Simple and non-tedious Easy to scale up commercially and economical Produces respirable particles required for deep lung penetration. This process does not alter the morphology and surface composition of the product. |
Not suitable for thermo labile materials. |
Spray freeze drying |
Suitable for thermo labile materials Maintains integrity of biotherapeutics and high production yield |
Process is time consuming |
Supercritical fluid |
Produces pure particles free from solvent residues Particles produced are suitable for inhalation. Green technology which can be recycled |
Problematic in scale-up. Uncontrolled particle size distribution and morphology. |
Recent Advances in Dry Powder for Inhalation
The advent of nanotechnology and advancement in nanoscience has led to various innovations in science and technology, including medicinal and pharmaceutical formulations. Nanotechnology has the ability to bring about a transformation in the scenario of medicines. For the past 15 years, there has been a great change in the formulation concepts, which has led to new regulatory challenges. Nanoparticulate formulations are found to be superior and very advantageous as compared to their conventional or macro particulate systems.
Amongst the existing formulation system, it is necessary to develop a formulation that will reduce the limitations produced by the existing system like instability, toxicity and irritation of the drug, deposition pattern and clearance mechanisms in the lungs, etc. This is possible by developing the nanoparticulate systems; they have the potential to produce the following effects (Boer, 2017).
-
Maintain uniformity in drug distribution in the alveoli
-
Influence the solubility of drugs
-
It affects and improves the pharmacokinetic profile of drugs by maintaining a sustained and prolonged release, which indirectly aids in the repeatability of dose, therapeutic efficacy and patient compliance.
-
Both macro and micro-molecules can be delivered, which are biocompatible and biodegradable in nature.
The nanoparticulate systems include microparticles or large porous particles, lipid-based systems like micelles, liposomes, proliposomes, solid lipid nanoparticles, nanostructured lipid carriers and polymeric nanoparticles.
Large porous particles/ Microparticles
Deposition of the particles in the upper respiratory tract, i.e. the mouth, throat or upper tracheal region leads to rapid elimination of the particles from the lungs by the various clearance mechanisms, which may lead to inefficient or inappropriate therapeutic efficacy for the intended use. Large porous particles are porous hollow structures having a large mean diameter of > 5 µm but a density of < 0.1 g/cm3. Although the geometric diameter of the particles is large due to their very low density, they represent the aerodynamic diameter equivalent to that of smaller particles (< 5 µm) having high density. The low density and large surface area of the particles lead to high dispersibility, which is an ideal characteristic required for pulmonary delivery. Large geometric diameter reduces the clearance by macrophage action, thereby enhancing the bioavailability and retention time in the lungs. Table 2 presents the summary of large porous particles prepared for pulmonary delivery and their methods of preparation.
Polymeric nanoparticles
The term microparticle (ranging within a size of 1-999 µm) comprise of microspheres and microcapsules. Microspheres basically relate to uniform spherical shaped particles consisting of a polymeric matrix and microcapsules comprises of an oily core surrounded by a thin polymeric membrane. Biodegradable microspheres are made up of natural and synthetic polymers and can incorporate hydrophilic and lipophillic moiety as well. In contrast to liposome, microspheres are more stable in nature both in-vitro and in-vivo, sustained release and longer retention time. Various polymers used are albumin, chitosan, polysaccharide, poly(lactic-co-glycolic) acid, poly(lactic) acid, poly(butyl cyanoacrylate) and poly(lactic-co-lysine graft lysine).
β- cyclodextrin
Cyclodextrin (CD) is nanomolecular inclusion complexes, which comprises of two or more entities in which one of the molecules is the host and second is the guest. Cyclodextrins are the result of the association of oligosaccharides and are formed of 6, 7 or 8 units of glucopyranose (α-, β-, γ- CD) respectively. β- Cyclodextrin seems to be more widely used cyclodextrin in pharmaceutical development because of its low production cost, size of the cavity and complexation efficiency with drugs. They can be used as a combination with other vectors. Earlier, they were used via pulmonary route for their pulmonary absorption promoter of peptides and proteins like insulin and calcitonin.
Lipid-Based Systems
Micelles
A successful drug carrier system needs to demonstrate optimal drug loading and release properties, long shelf-life and low toxicity. Colloidal systems, such as micellar solutions, vesicle and liquid crystal dispersions, as well as nanoparticle dispersions consisting of small particles of 10–400 nm diameter, show great promise as carriers in pulmonay drug delivery systems. Drugs can be trapped in the core of a micelle and transported at concentrations even greater than their intrinsic water solubility. A hydrophilic shell can form around the micelle, effectively protecting the contents. In addition, the outer chemistry of the shell may prevent recognition by the reticuloendothelial system, and therefore early elimination from the bloodstream.
A further feature that makes micelles attractive is that their size and shape can be changed. Chemical techniques using cross linking molecules can improve the stability of the micelles and their temporal control. Micelles may also be chemically altered to selectively target a broad range of disease sites. Amphiphilic macromolecules self-assemble to nanoscopic core/shell structures in an aqueous environment, namely polymeric micelles that can encapsulate water-insoluble drugs, proteins and DNA, and target therapeutics to their site of action in a passive or active way. Polymeric micelles share many structural and functional features with a natural transport system, e.g. virus and lipoproteins. The versatility of the synthetic chemistry in polymeric micelles is a bonus providing opportunities to design appropriate nano-carriers for individual delivery requirements. Development of nano-engineered polymeric micellar formulations that can address the problem of drug resistance corresponds to one focus of this drug targeting dosage form.
In this context, the effect of chemical manipulations on the encapsulation, release, bio-distribution and cellular interaction of the polymeric nanocarrier is assessed to select appropriate chemistries for optimized delivery of P-glycoprotein substrates to resistant tumors. Another focus of this nanometric dosage form is to improve the solubilization of a poorly water-soluble drug intented to be administered via the pulmonary route. The advantages of polymeric micelles include better stability than surfactant micelles, ability to solubilize an adequate amount of hydrophobic drugs, prolonged circulation times in vivo, and the capability to accumulate in the target organs. In this area, Jones and Leroux formulated beclomethasone dipropionate-loaded polymeric micelles directly administrable to the lung in nanoparticle sizes in an inhalation dosage form intended to for an effective treatment against asthma and COPD. This concept was able to be achieved since the polymeric micelles are able to evade the mononuclear phagocytic system due to their bulky hydrophilic outer shell and sustain the release of the drug (Jones & Leroux, 1999).
The polymeric micelles are very beneficial in delivering hydrophobic corticosteroids, such as beclomethasone dipropionate, for the treatment of chronic pulmonary obstructive disease since the inability of such drugs to penetrate through the
Drug |
Porogens |
Method of preparation |
Outcome |
Ref. |
---|---|---|---|---|
Meloxicam |
ammonium bicarbonate |
Spray drying |
The study was compared between non-porous and porous nanoparticles. The aerodynamic evaluation of porous particles showed a fine particle fraction (FPF) of up to 65.8% and emitted fraction (EF) reached 85.4% which was higher than that of non-porous particles |
|
Sodium Cromoglycate |
ammonium bicarbonate |
Spray Drying |
Production of large porous particles, good stability even at long storage periods, and excellent aerosolization performance with emitted dose of 84 % and FPF of 90%. |
|
Small interfering RNA (siRNA) |
None |
Spray freeze drying |
Moderate FPF of 30%, formulation revealed a lower density and smaller aerodynamic diameter (1-2µm) despite a MMAD of 4-5 µm. |
|
Budesonide |
L-Leucine Amino acid |
Spray Drying |
Morphology of the Formulated microparticle was seen as large, wrinkled and porous in nature. The emitted fraction obtained was 28- 45%. |
|
Budesonide |
poly(lactide-co-glycolide) poly(vinyl pyrrolidone) (PVP) poly(vinyl alcohol) (PVA) |
Lyophilization |
The optimised formulation showed EE of>60% and an aerodynamic particle size of ~6 μm (FPF>21%). |
|
Voriconazole |
Tert-butyl alcohol Pearlitol 160C |
Spray Freeze drying |
Fine particle fraction obtained was above 40% and higher dissolution rate in the initial 2 h. |
|
Budesonide |
PLGA Lactohale 200 Poly(vinyl alcohol) (PVA 205) poly(vinyl pyrrolidone) (PVP-k12) |
modified single emulsion (O/W) solvent evaporation method + Freeze drying |
The formulation showed a high geometric diameter of 9-20 μm but MMAD of 2 μm. The optimised formulation showed a slow release with the longest residence time of over 48 h. |
(Li, 2019) |
Doxorubicin Methotrexate |
D-mannitol Polyethylene glycol di-acid (PEGDA) |
Synthesis of magnetic-silica nanoparticles + Freeze drying |
Hollow structured nanoparticles were developed having a drug loading capacity of 48%. The aerosolised performance showed 22% of FPF. |
mucus layer to reach the target site, which results in the failure of treatment in the past. To rectify this disadvantage, drug-loaded polymeric micelles are strongly recommended to pass through the mucus layer associated with bronchial inflammatory diseases directly to their receptors in the epithelial cells. The same authors experimented and found the effect of the drug on its entrapment efficiency, drug-polymer molar ratio, in-vivo release and in-vitro inhalation deposition pattern and permitted them to conclude that micelles can be used as nanocarriers for.
Liposomes
Liposomes are spherical. Self closed structures composed of curved lipid bilayers which entrap part of the solvent, in which they freely float, into their interior. They may consist of one or several concentric membranes. These are extensively studied dosage form for inhalation. It can be used to deliver drugs of both hydrophilic and hydrophobic characteristics. Liposomes aid in the solubilisation of insoluble drugs, biocompatible with the lung surface, facilitate alveolar macrophage delivery and also maintain a sustained release (Liang, 2015; Wauthoz & Amighi, 2014).
This system was widely used to deliver the medicament by use of nebulizers; but it had some limitations of leakage of the entrapped drug. Recently, research reported the freeze-dried product of liposomal curcumin to be very efficient, low cytotoxicity and improved stability (Zhang & Chen, 2018). Though this system is very effective, the major drawback is the stability of the formulations; hence, to improve the stability issues, the liposomal formulations are converted to the dry powders by freeze-drying, spray drying, spray freeze drying, supercritical fluid technology, etc. Liposomes are composed of lipids, i.e. phospholipids, cholesterol and charge inducers (Bayindir & Yuksel, 2015). A phospholipid is the key ingredient in the formation of liposome and making it very effective in delivering the drug to the lungs.
The main component of lung surfactants is phospholipids. They are recognised as generally recognised as safe (GRAS) excipients for pulmonary delivery by the FDA. Phospholipid helps to potentiate drug pharmacokinectics by enhancing the drug permeability via the epithelium of the lungs (Wauthoz & Amighi, 2014). The main phospholipids used are phosphotidyl choline (primarily obtained from egg yolk or soyabean) and lecithin. Use of cholesterol is to provide proper arrangement and rigidity to the bilayered membrane. It also prevents the leakage of the entrapped drug by inhibiting the phase transition. To enhance the stability of colloidal carriers, charge inducers are added in order to cause repulsion between the particles. A few examples are stearylamine, phosphatidic acid, phosphatidyl glycerol, etc. (Bayindir & Yuksel, 2015). Table 3 represents the various formulation technologies and their effect on liposome-based DPIs.
Proliposomes
They follow the same concept as that of the prodrug. In the case of pro-vesicular system, the liposome formation will occur when it comes in contact with the biological fluids. Proliposomes (PL) enhance the long term stability, aids in large scale production, dosage homogeneity is maintained, and purity of natural phospholipids is not lost. PL is composed of phospholipid, cholesterol and carrier powder. These compositions can be altered with the use of charge inducers, bile salts, non-ionic surfactants, the polymer in order to attain the desired parameters such as stability, drug release and in-vivo efficiency (Bayindir & Yuksel, 2015).
Due to its advantages over the liposomal formulations, the researches have stated that one-step spray drying method can be implemented in the production of proliposomes of rifapentine-loaded proliposomes for the treatment of tuberculosis; the study conducted revealed 7 fold increase in both, the mean residence time and t1/2 (Patil-Gadhe & Pokharkar, 2014). Proliposomal ritonavir formulation for lymphatic targeting has been also developed and reported for its accelerated long term stability tests, in-vitro drug release and in-vivo pharmacokinetics (Ahammed & Narayan, 2017). PL can be prepared by methods such as film deposition, spray-drying, modified ethanol injection method, lyophilisation, supercritical anti-solvent technology, etc. The most widely used method to obtain proliposome for dry powder for inhalation is spray drying. (Rojanarat, 2011). Developed isoniazide proliposome by spray drying method to check the toxicity of proliposomes to respiratory-associated cell lines and its potential to provoke immunological responses from alveolar macrophages (AM). The study conducted proved that spray drying with to suitable carrier like mannitol can be used to achieve the desired range of particle size required for the deep lung penetration of the active constituent. Proliposome formulation did not show any toxicity or activation of any inflammatory cells (Rojanarat, 2011). A novel approach to produce antibiotic proliposome by coupling core carrier method with spray drying was performed by Ye t. et al. The study stated that proliposome is found to be more stable as compared to conventional liposome formulation.
Drug |
Key Ingredients |
Method of preparation |
Outcome |
Ref. |
---|---|---|---|---|
Folic acid Docetaxel |
Phosphatidylcholine Cholesterol |
Co-Spray drying method |
The comparative study of developed Docetaxel-loaded folic acid-conjugated liposomes with that of dry powder of the same produced by co-spray drying method showed increased cellular uptake via micropinocytosis and exhibited higher cytotoxicity against the tumor cells. Also, the tracheal administration resulted in 45 fold higher concentration as comparison to intravenous administration. |
|
Pyrazinamide |
1,2-dipalmitoyl-sn-glycero-3- phosphatidylcholine (DPPC) 1,2-distearoyl-sn-glycero-3-phosphoethanolamine N-(carbonyl-methoxy polyethylene glycol-2000) (DSPE-PEG2k) L-leucine |
Spray drying |
Spray dried particles showed a narrow size distribution (1.29–4.26 mm) with low residual moisture (<2%). The aerosolisation of spray dried powder was increased with high amount of DPPC (25% W/W) with FPF of 73±4.0%. |
|
Ciprofloxacin |
hydrogenated soy phosphatidylcholine cholesterol Sucrose magnesium stearate isoleucine |
Freeze- thaw + Spray Drying |
The formulated liposomal encapsulated ciprofloxacin nanocrystals (LECN) demonstrated a controlled release with a partical size of 1 μm, FPF of 66–70% and drug encapsulation efficiency of 71–79% |
|
Clarithromycin |
Soybean phosphatidylcholine (SPC) Cholesterol Trehalose Mannitol sucrose |
ultrasonic spray freeze drying |
The clarithromycin liposomal powder formulation had a high entrapment efficiency of up to 80%, high aerosol efficiency (emitted dose N 85%, fine particle fraction 43%–50%) and formulation properties were unchanged when stored at 60% RH at 25 ºC for 3 months. |
(Ye, 2017) |
Curcumin |
Soybean lecithin Cholesterol Mannitol |
Freeze drying |
The liposomal curcumin dry powder had a mass mean aerodynamic diameter of 5.81 μm and a fine particle fraction of 46.71%, suitable for pulmonary delivery. The uptake of curcumin liposomes by human lung cancer cells was markedly greater and faster than that of free curcumin. High cytotoxicity on Cancer cells and the low cytotoxicity on normal human bronchial was seen. |
|
Colchicine Budesonide |
1,2-Dipalmitoyl-sn-glycero-3- phosphoglycerol sodium (DPPG) Hydrogenated Soyaphosphotidylcholine (HSPC) Soyaphosphatidylcholine (SPC) cholesterol (CHOL) Mannitol |
Freeze drying |
The formulation demonstrated a sustained drug release up to 24 hours and a high entrapment efficiency of 97.89 - 98.6%. In the long term stability studies conducted the formulation was found to be stable. |
|
Gemcitabine-HCl |
HSPC DSPG mPEG2000-DSPE Cholesterol Trehalose L-leucine |
Lyophilization |
Aerosol performance study suggested favourable aerodynamic property of the formulation with FPF value of 56.12% and MMAD being 3.91 µm. Cell line studies showed a better uptake by human adenocarcinoma cells as compared to free drug. |
The stability studies were carried out at 60% RH and 25ºC for 3 months (Ye & Sun, 2018). Proliposome formulations, due to its benefits, find its application in the delivery to the lungs for the treatment of cancer caused by genes such as CY1PA1 (Zhang & Wang, 2019).
Solid Lipid Nanoparticles (SLN)
Solid lipid nanoparticles are stable colloidal carriers made of lipids solid at both room and body temperatures. They are formed of a solid lipid core stabilized by surfactant and containing the drug dissolved or dispersed. The rigidity of the lipid core is an important parameter which determines the rate of drug release. Despite the diversity of compounds involved in the preparation of solid lipid nanoparticles they are well tolerated by the lungs and are considered carriers of low toxicity. Compared to liposomes, solid lipid nanoparticles present the advantage of being more stable physically (e.g., during nebulisation). Solid lipid nanoparticles have been shown to sustain the release of different drugs in the lungs. (Li, 2019). prepared microparticles containing thymopentin-loaded solid lipid nanoparticles for pulmonary delivery. The microparticles showed the similar sustained release of the systemically acting peptide as free solid lipid nanoparticles in vitro. Following delivery to the lungs in rats, the microparticles resulted in sustained plasma concentrations of thymopentin over 48 h, whereas the unencapsulated peptide was cleared from plasma within 1 hr (Li & Sun, 2010). Having found that the direct intramacrophagic anti-tuberculosis therapy via dry powder inhaler devices necessary, Maretti et al., developed rifampicin-loaded SLN assemblies using melt emulsion technique followed by freeze-drying with the aim of achieving efficacious aerosolization. The research also focused to offer novel information regarding pre-freezing variables. The results gained showed a significant impact exerted by quick freezing combined with sample dilution before pre-freezing step without using cryprotectant on the respirable powder efficiency. The respirable powder achieved was >50% (Maretti, 2016). Recently, researchers have tried and have been successful in formulating hybrid solid lipid nanoparticles, i.e. solid lipid nanoparticles with added polymers. Rodenak et al. developed a strategy to potentiate the antimicrobial action of ofloxacin (broad-spectrum antibiotic). Formulation included incorporation of chitosan and eugenol into a lipid matrix by hot homogenisation/ ultrasonication method. This formulation resulted in enhanced bactericidal activity, minimization of MIC (minimum inhibitory concentration) from 6.1 to 16.1 fold of ofloxacin in encapsulated form, no toxicity in human cell models at 24 and 48 h exposure (Rodenak-Kladniew, 2019). The effect of L-leucine was studied by char et al. on the stabilization and aerodynamic characteristics of Spray-dried solid lipid nanoparticles. The experiment proved the ability of leucine as an important component required in maintain the particle size of spray drying and reconstituted solid lipid nanoparticles and improving the aerosolisation property (Yue-Xing & Fei-Fei, 2018).
Nanostructured Lipid Carrier (NLC)
Nano-structured lipid carrier is composed of a blend of solid lipid and liquid lipid in appropriate ratios. High drug loading is achieved with a less ordered lipid matix. NLC have benefits, because of its capability to produce controlled size, its sustained release, ability to retain for a longer period of time in the lungs, biocompatible and enhanced bioavailability (Patil & Deshpande, 2018). This leads to better patient compliance and reduced dosing frequency. Many researchers have exploited this area. Different types of NLC are formulated to achieve lung targeting or specific site targeting such as plain Nano-structured lipid carrier, multifunctional NLC (MNLC), PEG-NLC, etc. These formulations are basically administered via nebulisation or as aerosolization (Jaafar-Maalej & Andrieu, 2011; Jyoti & Kaur, 2015; Taratula & Kuzmov, 2013). With respect to dry powder for inhalation, Patil-gadhe et al. developed and characterised montelukast loaded NLCs. It was formulated by melt-emulsification-ultrasonication method followed by freeze-drying technique to obtain DPI. Mannitol was used as a cryoprotectant and carrier. NLC produced showed high % entrapment efficiency (> 95%), high safety and efficacy levels when investigated via in-vitro cytotoxicity assay and pharmacokinetic evaluation parameter, respectively (Patil-Gadhe & Pokharkar, 2014). Another study conducted by the same authors involved the use of rosuvastatin for the treatment of airway remodelling in COPD. Here, rosuvastatin dispersion was formulated by hot melt-emulsification- ultrasonication method and then converted to respirable particles via lyophilisation using 5% mannitol as cryoprotectant. The mass median aerodynamic diameter (MMAD) and fine particle fraction (FPF) achieved was <3 µm and >90% respectively. The improvement in bioavailability was found to be enhanced by 35 fold. The lipid nature and smaller particle size aided in bypassing macrophage clearance leading to higher targeting factor (Patil-Gadhe & Pokharkar, 2016).
Conclusion
Pulmonary delivery of pharmacologically active drugs has been extensively investigated as a very promising alternative route for either local or systemic treatment. The successful administration of any therapeutic agent via DPI mainly depends on four mutually dependent parameters, i.e. the formulation, the metering system, inhaler device and patient compliance. The merits of DPIs like simple and cheap devices, robust, portability and ability to produce fine particle fraction and de-aggregation upon actuation by breath. Until now, no marketed formulations are available with a controlled release feature for pulmonary delivery. To accomplish this, various formulation aspects like large microporous particles, lipid-based system and polymeric nanoparticles provide a promising approach to overcome the disadvantages caused by the conventional formulation. Various methods and their modifications can be utilised for the production of respirable particles required for the deep lung penetration with a size range of 1- 5µm.