Importance of Nanoparticulate systems in emerging Biomedical research - An update
Abstract
Nanotechnology is the branch of science and engineering accomplished by design, synthesis, characterisation, and application of materials or devices in the nanometer scale. It permits identification, development and establishes the use of material frameworks, devices and technologies withs unique properties and functions from their structures at the Subatomic, atomic, molecular as well as supermolecular levels. In recent decades, nanotechnology has attracted unparalleled attention in broad areas of research. It has been used in various fields like Engineering and Technology, Material Science, Agriculture, Medicine, Pharmacy, Biotechnology and other areas of Life science. Nanoparticles have been playing a promising role in the advancement of diagnosis and therapeutics of many health issues. They are providing the way for new approaches of drug delivery to target specific sites and achieve enhanced bioavailability. In this review, we provide an overview of the use of Metallic nanoparticles, lipid-based nanoparticles, Charge reverse nanoparticulate systems, Carbon nanotubes, Magnetic, Controlled release nanoparticles, Herbal nanoparticles etc., in Various biomedical fields like Drug delivery, Gene therapy, Tissue regeneration, Implants, Diagnosis, Imaging and treatment of various diseases. Even though numerous nanoparticulate systems were developed, only a few of them are transformed into practical use in clinical environments. Hence, focus on the development and commercialisation of nanoparticulate system is entailed.
Keywords
Diagnosis, Drug delivery, Gene Therapy, Herbal, Nanoparticles, Tissue regeneration
Introduction
Nanotechnology enables the identification, development and establishes the use of material frameworks, devices and technologies withs unique properties and functions from their structures at the Subatomic, atomic, molecular as well as supermolecular levels (Roco, 2003). On many fronts, the field of nanotechnology is undergoing explosive growth. Nanotechnology plays a vital role in various studies like site-specific, targeted drug therapy, detection of disease in the early stage and many more (Sahoo & Labhasetwar, 2003). It increases the solubility of poorly water-soluble drugs, prolongs the half-life of the drug, releases drugs at a controlled pace or in an environment-friendly manner and thus lowers the frequency of administration and provide medications intended to mitigate systemic adverse effects. In a combination of drug therapy, it releases all the drugs concurrently to shows synergism and also prevents drug resistance (Zhang et al., 2008). In recent decades, nanotechnology has attracted unparalleled attention in wide areas of science. Since a few years, there has been a drastic increase in the number of nanoparticle medications and also clinical studies on therapeutic nanoparticles (Zhang et al., 2008). Toxicological considerations and ethical issues on nanoparticles have been casting a long shadow over the promising future of this emerging technology (Sanvicens & Marco, 2008). This article presents an overview of current applications and development of the nanoparticles in Pharmaceuticals and other allied areas of health Science.
Synthesis of Nanoparticles (NPs)
Nanoparticles can be produced by physical, chemical and biological methods to impart unique characteristics at a nanoscale (Nadaroglu, Güngör, & İnce, 2017). Preparation of nanoparticles involves two key approaches (Table 1). Firstly the "top-down" approach where large solid particles are divided into small parts by applying external strength. Several physical, chemical and thermal techniques are used in this method to provide the energy needed for the creation of nanoparticles. Secondarily the ‘bottom-up’ approach, where gas or liquid atoms or molecules are collected and combined to prepare nanoparticles. Both the methods are mutually beneficial as well as disadvantageous (Iravani, 2011).
Nanoparticles in Drug delivery
Lipid-based solid nanoparticles are highly permeable to cellular and viral reservoirs with significant improvement in their solubility and bioavailability (Pathak & Yashwant, 2019).
Carbon nanotubes can adsorb or interact with a broad range of therapeutic and diagnostic agents (Drugs, DNA, Vaccines, Antibodies, Bio-components, etc.) due to their large surface area. They can be used effectively in drug therapy and medical devices. They have proved to be an excellent vehicle for the delivery of drugs directly to targeted cells with no first-pass metabolism (He et al., 2013).
Charged-reverse nanosystems are employed with space-controlled, temporal and dosage-controlled approaches in the delivery of anticancerous agents. These nanoparticles discharge their drug after receiving particular stimuli and modify their surface charges. They are activated in response to protonation, cleavage or molecule conformity changes these nanoparticles delays, drug clearance from systemic circulation (Guo, Chen, Tian, & Chen, 2016).
Polymeric and lipid-based nanoparticles, carbon and rigid inorganic nanoparticles and also specific ligand coupled nanoparticles can turn off the effects of Multi-Drug Resistance genes and other oncogenes by targeting siRNAs (Aghamiri, Jafarpour, Malekshahi, Gomari, & Negahdari, 2019).
The development of protein-based noninfectious nanoparticles derived from the capsids of the virus is emerging today as a virus-like particle for various biomedical applications (Rohovie, Nagasawa, & Swartz, 2017). Metallic nanoparticles have gained a property that enhances therapeutic efficacy, controlled distribution, improved drug localisations, and reduced toxicity (Iqbal, Halasz, & Bhatia, 2018). Various Controlled release and extended-release nanoparticle formulations like pH-Triggered Controlled Drug Release Nanoparticles, Electric Field Responsive Nanoparticles, Controlled drug release hydrogel nanoparticle networks, Porous hollow controlled-release nanoparticles, Solid lipid-based controlled-release nanoparticles, Extended-release polymeric Nanoparticles are developed to overcome several complications (Patra et al., 2018).
Physical |
Chemical |
Biological |
---|---|---|
Approach- Top Down |
Approach- Top Down Bottom-up |
Approach-Bottom up |
Arc Discharge |
Chemical reduction of Metal Salts |
Using Microorganisms |
Ion Implantation |
Macro emulsion method |
Using industrial and agricultural wastes |
Inert gas Condensation |
Electrochemical method |
Using algae |
Milling |
Pyrolysis |
Using Enzymes and Biomolecules |
Spray Pyrolysis |
Sonochemical method |
Using Plant and their Extracts |
Ultrasonic fields |
Sol-gel Process |
|
Electron beam Lithography |
Co-Precipitation method |
|
Mechanical grinding |
Phytochemical Method |
|
Vapour Phase Synthesis |
Solvothermal Synthesis |
|
Photochemical reduction |
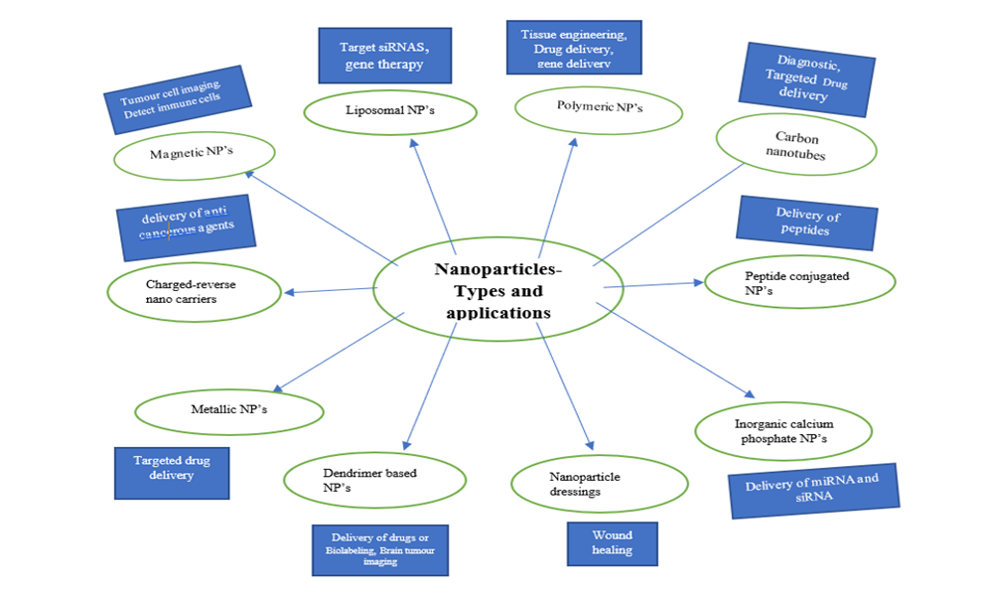
Nanoparticles in Imaging and Diagnosis
The application of nanotechnology in diagnosis and imaging of diseases has been progressing day today. It led to the development and advancement of biomedical products. Popular nanomaterials for biomedical use include liposomes, polymer micelles, graphene, carbon nanoparticles, quantic points, Ferro-ferric oxide nanoparticles, and superparamagnetic nanoparticles (Kong et al., 2017).
The magnetic nanoparticles are used as contrasting substances in magnetic resonance imaging (MRI), colloidal mediators for tumour cells magnetic hyperthermia and active components for drug delivery platforms. These applications have drawn attention to emerging Medical and Pharmacological research (Duguet, Vasseur, Mornet, & Devoisselle, 2006).
Magnetic nanoparticles that work on the principle of magnetic force-based microfluid immunoassay are successfully used to detect immune cells IgG in rabbit and mouse models (Sung & Park, 2005).
Bio conjoint nanoparticles can be used in highly sensitive biomolecular assays and in-vivo molecular applications by linking nanoparticles to bio-refined ligands such as tiny organic compounds, proteins or oligonucleotides (Kairdolf, Qian, & Nie, 2017).
Circulating vascular nanoparticles can have direct access to selected molecular disease markers on endothelium and are ideal for vascular targeting facilitated by geometrically enhanced multivalent attachments on the vascular target (Atukorale, Covarrubias, Bauer, & Karathanasis, 2017).
Liposomal nanoparticles have been developed to visualise placental margin and placental/uterine interface by utilising blood pool contrasting medium that does not traverse a placental barrier but offers a promising method to evaluate clinically invasive placental pathologies (Ghaghada et al., 2017).
The dendrimer conjugated magnetic NPs have been used as an MRI contrast agent that shows an apparent generation of heat in the alternating magnetic field and a favourable property for T2-weighted imagery. These two applications with covalent conjugation chemistry ensure organic shell stability to avoid cellular transportation desorption. These nanoparticles can also be applied in the delivery of drugs or biomarking by conjugating it with high loading terminal amine groups (Esmaeili et al., 2019). Functionalising NPs develop Dendrimer-based nanoparticle imaging techniques for magnetic, and fluorescence imaging and also dendrimer-based optical/paramagnetic nanoprobe can bypass the Blood-brain barrier and capture high-sensitivity brain tumour images (Posadas, Monteagudo, & Ceña, 2016).
Nanoparticles in Tissue regeneration
In years, nanotechnology has gained increasing attention for improving current approaches to tissue and organ regeneration. In particular, nanoparticles have interesting features to facilitate regenerative medicine (Rijt & Habibovic, 2017). Nanoparticles allow a unique amalgamation of strategies for the engineered regeneration of tissues such as scaffold modification, cell labelling and delivery of drugs and genes (Filippi, Born, Felder-Flesch, & Scherberich, 2019). The effectiveness of wound care can be significantly improved by using biocompatible nanoparticle-based dressings which regenerates the functions of skin quickly and safely by releasing the bioactive drugs on a regulated and sustained basis (Berthet, Gauthier, Lacroix, Verrier, & Monge, 2017).
Magnetic NPs have been used to stimulate differentiation of stem cells without limiting proliferation, for orthopaedic applications. Nevertheless, careful consideration has suggested the use of MNP-associated active agents to differentiate between certain lineages (Wimpenny, Markides, & Haj, 2012). The 3D printed drug conjugated hydrogel channels, with its tailored nanosize, form and structure promote nerve regeneration by releasing the drug in a sustained manner (Tao et al., 2019). Nanoparticles containing endothelial growth factor improves cardiac functioning and prevents remodelling of left ventricles in the heart of mice with experimentally-induced Myocardium infarction (Oduk et al., 2018). Aqueous solutions of nanoparticles with rapid release and adhesion properties are used for highly complex clinical conditions such as wounds, bleeding and tissue repair in various organs like spleen, liver, heart or lung procedures (Meddahi-Pellé et al., 2014).
Nanoparticles are also taking part in dental benefits by regenerating dental tissues. These particles are used in the therapy of periodontal defects, regeneration of the jaws and lips and probably even it can be utilised in the recovery of lost and damaged teeth (Subramani, Waqar, James, & Hartsfield, 2013). Ultraviolet-reactive, rapidly cross-linkable scaffold integrated with kartogenin-loaded nanoparticles demonstrated its potential role in cell homing, particularly for the recruitment of endogenous host cells, including mesenchymal stem cells derived from bone marrow and Synovium (Shi et al., 2016). The calcium phosphate nanoparticles can smoothly deliver miRNA and siRNA to the target cell by forming stable complexes and allow its discharge to the cytoplasm (Levingstone, Herbaj, Redmond, McCarthy, & Dunne, 2020).
Nanoparticles in Gene Therapy
Due to rapid development of nanotechnology and genomics, nanoparticle-based gene therapies were built over the last two decades to treat different diseases by overcoming structural-functional issues, anatomical barriers, stability and availability of nuclear acids, immune reactivity and delivery paths (Chen, Liu, & Jiang, 2016). It has excellent ability to absorb, focus and secure DNA, which can be ascribed as a primary reason for the adsorption and activity of the exogenous gene vector (Sun, Liu, Huang, Tian, & Hu, 2014). The safer alternatives for the delivery of genes are biodegradable polymeric nanoparticles, but their use in-vivo has been limited due to its low efficacy (Mangraviti et al., 2015).
Angiopeptide-conjugated dendrigraft poly- L-lysine (DGL) based nanoparticles can mediate gene expression in-vitro and in-vivo. Intravenous administrations of these nanoparticles in multiple doses improves the recovery of rotenone-induced parkinsonism in rat models. These strategies can be applied for longstanding non-invasive gene therapy of neurodegenerative diseases (Huang et al., 2013). The in-vitro and in-vivo studies of oligonucleotide conjugated with chitosan-derived nanoparticles can significantly allow C-siPlk1 entry into cancer cells, and they are highly efficient in the down-regulation of Plk1 gene (Plk1) to show potent tumour inhibition (Zhang et al., 2016). Safe liposomal nanoparticles are designed to address challenges such as systemic obstacles (especially serum degradation), Reticulo endothelium system clearance, off-target effects, poor stability, endosomal escapes and toxicity in human gene therapy (Zylberberg, Gaskill, Pasley, & Matosevic, 2017).
Herbs in Nanoparticles
Phytonanotechnology has handed over new ways for nanoparticle synthesis, which is environmentally friendly, simple, fast, stable and economical. Its benefits include Scalability, biocompatibility and use of water as a solvent (Huang et al., 2013). Thus, plant-derived nanoparticles produced from readily available plant materials are adopted to meet the high demand for biomedical and environmental applications (Singh et al., 2016).
Mono-functional and bifunctional herbal nanoparticles have been synthesised for different applications like antibacterial, antianemia including cancer (Raghunandan et al., 2011). Herbal nanoparticles conjugated with the metal oxides are synthesised by a variety of green synthesis techniques, and it gained more significant importance for their nontoxic nature. However, the extent of herbal nanoparticle commercialisation is not satisfactorily.
Various types of Nanoparticle and their specific applications are summarised (Figure 1).
Conclusion
Nanoparticles have been playing a promised role in the advancement of diagnosis and treatment of several illnesses. They are paving the way for new approaches of drug delivery to target specific sites in treatment. Although many types of nanoparticulate systems were developed, only a few of them are translated into clinical environments. Treatment with herbal nanoparticles is safe and effective. However, they are underutilised, and their commercialisation is not progressive. Therefore, these lacunae open a new arena for research, development and commercialisation of herbal nanoparticles. The nanomedicine spectrum is broad, and even wider because several numbers of materials can be nano-sized. Simultaneously, Toxicological concern of a nanoparticle is a major drawback and yet to be solved efficiently.