Critical review on role of Microbiota transfer therapy in Autism spectrum disorder
Abstract
Autism spectrum disorder (ASD) is a developmental disorder that affects behaviour and communication. It can be diagnosed at any age, although the symptoms generally appear in the first two years of life. Autism is also known as a "spectrum" disorder because there is wide variation in the severity and type of patient experience. Intestinal dysbiosis remains a risk factor for several neuron-developmental disorders and physiological diseases such as ASD. The gut microbiome in human influence the total metabolomic profile and thus has an impact on the overall health of human. The brain-intestinal axis concept demonstrates the interaction between the brain and the diverse gut microbial population. Recent findings show that ASD symptoms in affected individuals are linked with the altered intestinal microbiome. The current treatment modality for the symptoms of ASD are limited to Applied Behaviour Analysis (ABA) and FDA approved medications like Risperidone and Aripiprazole. Individuals with ASD appear to be susceptible to adverse effects of these medications. Current accumulating studies indicate that Microbiota Transfer Therapy (MTT) is a promising technique for treating ASD. MTT involves in vitro batch culture of gut microbiota, which is viewed as a quick and accessible method. It has provided encouraging results by improving ASD-related gastrointestinal (GI) and behavioural symptoms in affected individuals. The present review focuses on the link between gut dysbiosis and ASD, analyses the available clinical studies related to various treatment options and describes the role of MTT as a promising therapy of bringing back the healthy gut microbiota composition in treating ASD.
Keywords
Autism spectrum disorder, Chelation therapy, Faecal microbiota transplantation, gut dysbiosis, gut microbiome, Microbial transfer therapy, Probiotics
Introduction
Autism spectrum disorder (ASD) is a developmental disorder that affects communication and behaviour. It is a "developmental disorder" as the symptoms generally appear in the first two years of life. Autism is also known as a "spectrum" disorder because there is a wide variation in the symptoms the affected individual's experiences. The current prevalence rate for ASD is 6 per 1000 children. ASD is characterized by repetitive and unusual sensory-motor behaviour and interests that can be diagnosed at any age. People with ASD may communicate, interact, behave, and learn in different ways from most other people. The learning, thinking, and problem-solving abilities of people with ASD can range from gifted to severely challenged (https://www.ninds.nih.gov/Disorders/Patient-Caregiver-Education/Fact-Sheets/Autism-Spectrum-Disorder-Fact-Sheet). ASD can be of substantial economic burden, due to the provision of support to ASD affected individuals who cannot function independently. Studies indicate that ASD results from early altered brain development and neural reorganization. As there are no specific biomarkers, the diagnosis is made on the basis of behaviour aspects. The American Psychiatric Association's Diagnostic and Statistical Manual of Mental Disorders (DSM)-5 criteria (2013) prepared standard classification criteria for mental disorders, which can be used by health professionals for diagnosis. They characterized the ASD spectrum diagnosis based on the two domains - social communication, and restricted, repetitive, or unusual sensory-motor behaviours. DSM-5 perceives that ASD can be accompanied by genetic disorders such as fragile X syndrome, attention-deficit hyperactivity disorder [ADHD] and other comorbidities like anxiety, depression, phobias, irritability, aggression, seizures, sleep difficulties, gastrointestinal disorders, mitochondrial dysfunction, and immune system abnormalities (Rossignol & Frye, 2012). There are various difficulties in identifying successful treatment methods for ASD, including small sample size, lack of participants and the use of outcome measures that are not uniformly adopted.
The gut microbiota is recognized for its decisive role in an individual's health. Microbiota refers to the whole population of microorganisms that colonize a specific area; and incorporates not only bacteria but also other microbes such as fungi, archaea, viruses, and protozoans. In a healthy human, the gut microbiota is in homeostasis to prevent the colonization of pathogenic microbes. A few factors that assume a part in forming the typical gut microbiota has been depicted in Figure 1.
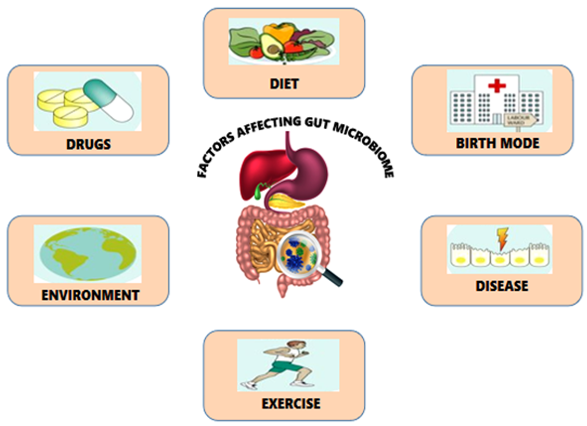
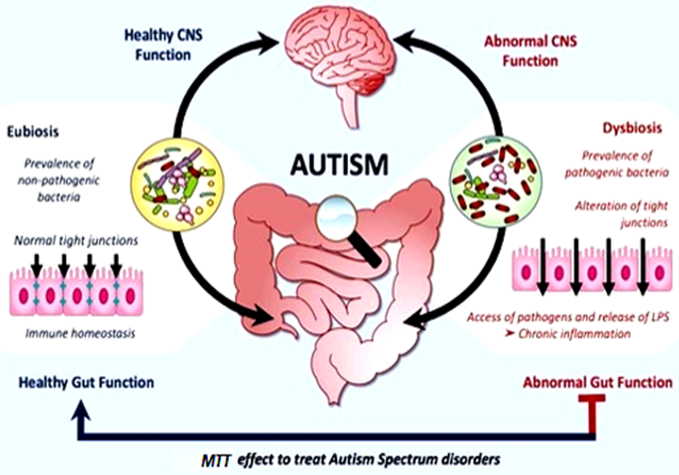
This “microbial organ” (gut) possess approximately 800 to 40,0000 species of microbes. These microbial communities represent a flourishing microbial bioreactor, delivering fundamental metabolites, hormones and enzymes required for nutrient metabolism, maintaining gut integrity, immunomodulation, drug and xenobiotic metabolism, and protection against pathogens in the host (Jandhyala, 2015). Several mounting evidences on the significant role of gut microbiota in maintaining human health status are provided by the US Human Microbiome Project (HMP), European Meta-genomics of the Human Intestinal Tract (Meta HIT).
Recent studies imply that the gut-brain axis (a bidirectional network of communication between the brain and the gut) has a vital role in the prevention and treatment of neuropsychiatric disorders like ASD. As gastrointestinal symptoms prevail in all cases of ASD, it is now evident that gut microbial dysbiosis remains a significant factor for the severity of ASD symptoms. Gastrointestinal (GI) symptoms, including constipation, diarrhoea which is caused due to the presence of pathogenic microbes in the gut, are correlated with behavioural aspects noted in ASD such as anxiety, self-injury and aggression. In particular, dysbiosis is associated with increased intestinal permeability to neurotoxic peptides of bacterial origin. These microbial metabolites released from pathogenic organisms disrupt the neural mechanisms and functioning of CNS, which eventually lead to neurodevelopmental disorders such as ASD and neuropsychiatric disorders, as shown in Figure 2. (Abdellatif, McVeigh, Bendriss, & Chaari, 2020).
While many children with ASD are provided with behavioural therapies as a treatment modality, recent studies have found negligible proof to exhibit their efficacy. Medications used to suppress gastrointestinal symptoms do impart positive results, but affected individuals remain susceptible to adverse side effects of the given medications (Kent et al., 2013). Considering the connection between the gut and brain, researchers have now focused on the therapeutic modulation of the gut microbiome to get beneficial results. It has led to the use of an alternative therapy called "Microbial transfer therapy" (MTT) for ASD treatment (Kang & Adams, 2017).
Microbiota transfer therapy (MTT) is the process of transferring beneficial bacteria and other microbes from a healthy individual into another individual. It has been utilized tentatively to treat other gastrointestinal diseases, including constipation, colitis, irritable bowel syndrome, Clostridium difficile infection and neurological conditions, such as multiple sclerosis, Parkinson's disease and ASD. The different MTT strategies used are Prebiotic / Probiotic / Synbiotic administration, phage therapy, Bacterial consortium transplantation and Faecal microbiota transfer therapy (FMT). Faecal microbiota transplant (FMT) was found to be the most promising strategy in MTT when compared to other techniques available. FMT uses the concept of bacterial interference, which employs beneficial bacteria obtained from the faeces of a healthy donor to displace pathogenic organisms in the recipient by competitive niche exclusion. It was found that faecal filtrate components such as metabolites, bacteriophages and bacterial enzymes serve as the key mediators of FMT’s efficacy, rather than the microbial population itself (Kang et al., 2017).
In 2019, the FDA granted "Fast track" status to the use of microbiota transplant therapy for ASD due to the procured promising results. Hence, it is evident that MTT intervention potentially manipulates the dysbiotic intestinal microbial communities into a beneficial composition and thereby provides successful treatment for the broad spectrum autism disorder (https://www.neurologyadvisor.com/topics/pediatric-neurology/microbiota-transfer-therapy-gets-fast-tracked-for-asd-treatment/).
Autism spectrum disorder
Autism spectrum disorder (ASD) is a neurological developmental disorder that has a profound impact on an individual's communication and social behaviour. It is called a "Developmental disorder" because the symptoms occur at the first two years of life and can be diagnosed at any age.
https://www.nimh.nih.gov/health/statistics/autism-spectrum-disorder-asd.shtml It is also known as "Spectrum disorder" as the affected people exhibit a wide varied range of symptoms (https://www.ninds.nih.gov/Disorders/Patient-Caregiver-Education/Fact-Sheets/Autism-Spectrum-Disorder-Fact-Sheet). ASD is widely accepted as a complex, pervasive, heterogeneous condition with multiple sub-types, etiologies and developmental trajectories. As it occurs in all kinds of racial groups anonymously, its effects differ based on many aspects such as environment, health status, exposure, diet and medical history https://www.nimh.nih.gov/health/statistics/autism-spectrum-disorder-asd.shtml.
Current treatment modalities
The treatment modality for ASD affected individuals varies across different countries and regions. The wide range of treatment modalities in use are:
Parent-mediated interventions
The treatment involves coaching the parent or caregivers on how to interact with children affected with ASD. Such learning develops joint engagement and encourages children to take more initiative.
Behavioural enhancement interventions
The most popularly known treatment which falls under this category is “Applied behaviour analysis" (ABA). It generally involves teaching or improvising the developmental skills like imitation, communication, cognitive tasks and adaptive skills in an affected individual by a therapist. The treatment process also involves several testing aspects in affected individuals through questionnaires and interviews.
Other treatments which use the same treating protocol are pivotal response treatment (PRT), joint attention symbolic play and engagement regulation (JASPER) and early social interaction (ESI) (Schreibman et al., 2015).
Pharmacological interventions
Atypical antipsychotic drugs are used to treat ASD symptoms in affected individuals. The most widely used medications are Risperidone and Aripiprazole, which improve symptoms of irritability and agitations like anger, self-injury and disruptive behaviour (Kent et al., 2013). Other medications like Methylphenidate, Atomoxetine and Guanfacine are used to treat Attention deficit hyperactivity disorder (ADHD) symptoms in autism spectrum disorder (Sturman, Deckx, & Driel, 2017). These medications have shown very low efficiency and have also caused adverse events in individuals affected with ASD.
Other interventions
Pilot studies that concentrate on utilising nutrient enhancements/vitamin supplements such as folic acid and sulforaphane have uncovered some believability in ASD treatment, yet the further substantial proof is required (Singh et al., 2014).
Chelation therapy, which involves the removal of heavy metals (Mercury, Lead, Arsenic) from the body and Hyperbaric oxygen therapy, which increases the supply of pure oxygen to the brain that eventually improves mitochondrial dysfunction, have also been tried as one of the treatment methods for ASD. Studies indicate that the use of these therapies leads to adverse effects such as slow metabolic activity, hypocalcaemia, anaemia, liver damage, seizures, hypotension, renal impairment and sometimes death, which outweigh its proven benefits (James, Williams, Silove, & Stevenson, 2015).
Recent interventions
New studies feature the significance of bacterial organisms in the human gut for the determination and treatment of ASD. Researchers noticed the consequences for ASD indications following the utilization of a progressive methodology, known as "Microbial transfer therapy" (MTT) (Kang et al., 2019).
In this method, gut microbiota from healthy donors has relocated into ASD affected individuals over 7-8 months, which produced marked microbiome changes in the ASD gut, improving both gastrointestinal and social conduct indications of the illness. Astoundingly, the improvement continued for a longer duration after completion of the examination(https://www.news-medical.net/news/20201115/MTT-procedure-improves-gastrointestinal-behavioral-symptoms-in-ASD-children.aspx).
Role of gut microbiota
The second-largest surface in our body is the Gastrointestinal tract, with an area ranging from 250-400m2. The gut microbiome persists around 1013 - 1014 microbial cells with a 1: 1(microbial: human) cells ratio. It comprises almost close to 100 times the number of genes in humans. Human and their interconnection with microbes fall under the concept called “ Holobiont". The gut microbiota is significantly recognized for its important decisive role in an individual's health. In a healthy human, the gut microbiota is in homeostasis to prevent the colonization of pathogenic microbes. When dysbiosis occurs, it leads to disease conditions in a host such as inflammatory, neurodegenerative, respiratory, hormonal impairment and cardiovascular diseases. The beneficial microbiome produces enzymes needed by the host to control its metabolism and physiological functions like shaping the epithelium, reaping energy, regulating host immunity and protecting from pathogens (Gensollen, Iyer, Kasper, & Blumberg, 2016).
Interestingly, study analysis in the gut microbiota of 33 samples from different nationalities threw light upon the fact that it is composed of three major enterotypes: Bacteroides, Prevotella and Ruminococcus. It was found that out of nearly 200,000 rRNA sequences in GENBANK, 1822 were from Human gut origin (Backhed, 2005). From a case-control study (Eckburg, 2005), phylogenetic analysis of gut microbial diversity in five healthy individuals revealed that out of 13,355 prokaryotic rRNA sequences, 11,831 were Bacterial species and the remaining 1524 were Archaeal species. All 1524 archaeal species are of a single phylotype: Methanobrevibacter smithii, while there occur 395 different bacterial phylotypes with 301 belonging to Firmicutes phylum and 65 to Bacteroidetes phylum. All these statistical findings were made by identifying and characterizing the gut microbes through culture techniques before the introduction of the revolutionizing "Culture-independent techniques" such as High-throughput sequencing of 16SrRNA, Metagenomics, Metaproteomics, Next-generation sequencing platform using Qiime software suite/Mothur suite (Browne et al., 2016).
Several studies suggest that the gut microbiome has an impact on infants growth and development by involving them in growth hormone production. The microbial gut flora gets affected by many major factors such as age, lifestyle, undernutrition, exposure to pathogens, antibiotics vulnerability, maternal health status, types of complementary feeding and life events. Through scientific evidence, it is clear that microbial exposure in infants from maternal microbiota starts before delivery. A study by (Bäckhed et al., 2015) suggests that vaginally delivered infant’s faecal microbiota (FM) are 72% similar to their maternal FM and are dominated by Lactobacillus and Prevotella organisms; While, FM of infants born by C-section delivery were similar to their maternal FM only by 41% and are dominated by Streptococcus, Corynebacterium, and Propionibacterium spp. Development of infant’s gut microbiota occurs at different periods:
-
Prenatal factors - Placental exchange during gestation
-
Neonatal factors - Mode of delivery at the time of birth
-
Postnatal factors - Location, Environment, Host interaction, weaning process. Feeding habits in infants plays a crucial role in altering the gut microbiome; Enterococcus, Bacteroides, Clostridia and Streptococcus genus occupy in formula-fed infants, while Bifidobacterium, Lactobacillus spp. Dominate in breast-fed infants as they possess the ability to break down Human Milk Oligosaccharides (HMO). The daily diet also plays an important role in gut microbiota, as organisms of the Firmicutes phyla, Ruminococcus bromii and Roseburia genus prevailed in individuals having plant based diets rich in insoluble carbohydrates. In individuals consuming an animal-based diet, gut flora is generally rich in bile tolerant organisms such as Alistipes spp and Bilophila spp.
Significant role of gut microbiome
Gut microbiome performs a plethora of activities essential for the host's functions, such as protection against pathogens, immune-system modulation, fermentation of non-digestible dietary fibres, anaerobic metabolism of peptides and proteins, interaction with the host's circadian clock and biotransformation of xenobiotics.
Gut-brain interlink
The gut microbial flora has a great impact on the brain's functionality. Microbes are the only source for producing Vitamin B12, hence deficiency of this leads to haematological and neuropsychological disorders. In the study by (Xiong et al., 2004), experiments with germ-free mice and normal healthy mice revealed that the gut microbes in healthy mice produce Short-Chain Fatty Acids (SCFA), which acts upon GPR41to produce Leptin in adipocyte cells. This Leptin, in turn, leads to gut-brain axis immune-inflammatory crosstalk. Interaction between gut microbes and brain is depicted in the study by (Mohajeri, Fata, Steinert, & Weber, 2018), wherein they indicate that the host's immune cells along with the gut microbes produce Immunomodulators, Cytokines, SCFA and Neurotransmitters [ACTH- Adrenocorticotrophic hormone, CRF - Corticotrophin releasing hormone] which bi-directionally communicates with the brain and has an effect on its function. The gut-brain communication occurs via the Vagus nerve, Endocrine nervous system and Central spinal afferent, which receive and send signals to the brain in a bidirectional manner, as shown in Figure 3. Studies have shown that Campylobacter jejuni species has an effect in decreasing the anxiety and exploratory behaviour of the host by activating the immuno-neural responses. The Lactobacillus species also has a great influence on the brain functions, as Lactobacillus helviticus R0052 is involved in CNS neuron functions in the hippocampus and Lactobacillus reuteri (DSM17938) is involved in visceral nociceptive gut neurons function (Gilbert et al., 2013). In addition, Bifidobacterium infantis stimulates tryptophan levels in plasma and thus improves stress associated gastrointestinal dysfunction and gut-brain axis regulations. Organisms such as Lactobacillus brevis, Bifidobacterium adolescentis, Bifidobacterium dentium, Bifidobacterium infantis are involved in the production of GABA (γ - aminobutyric acid), which is a neurotransmitter. Even the abundantly present organisms like E.coli, Bacillus and Saccharomyces species have participated in the nor-adrenaline hormone synthesis, which interact with brain metabolism and neuronal signalling (Lyte, 2013).
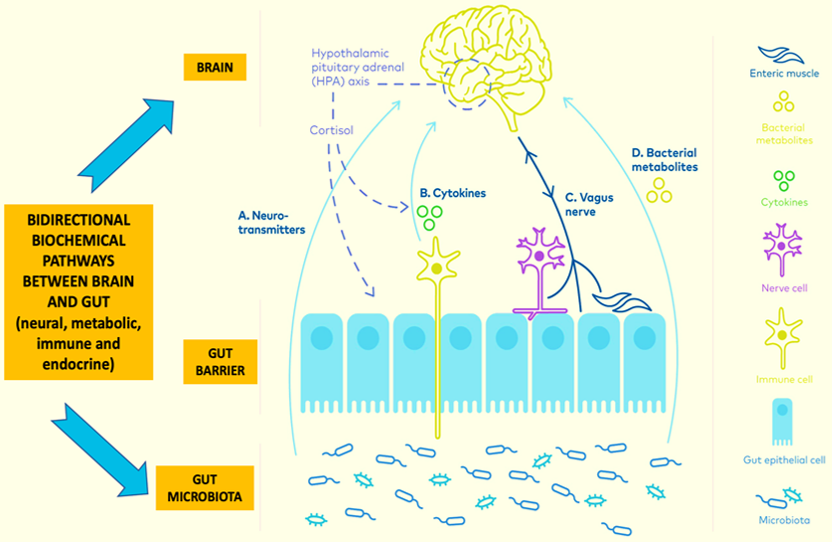
Antimicrobial protection and immunity
The beneficial microbe population in gut like Clostridium perfringens, Clostridium scindens, Bacteroidetes and Firmicutes convert the primary bile salts into secondary bile acids such as lithocholic acid (LCA) and deoxycholic acid (DCA) through microbial bile salt hydrolase (BSH) enzymes. The organisms Lactobacillaceae, Ruminococcaceae, and Desulfovibrionaceae, along with the production of secondary bile acids, also has the potential to elevate human Anti-microbial peptide - IL37 (Khanna et al., 2017). These microbial metabolites inhibit the growth of infectious pathogens in the gut. Most of the gram-negative bacteria activate intestinal dendritic cells, which in response stimulate the production of secretory IgA (s-IgA). These s-IgAs coat the gut microbiota and prevent the invading of pathogens. Bacteroides thetaiotaomicron and Listeria innocua are the key species in inducing Anti-microbial proteins (AMP) in host Paneth cells through pattern recognition receptor - microbe associated molecular patterns activate signal pathways to produce AMP, IgA and Mucin glycoproteins. The commensal microbes such as Bacteroides fragilis enhance and support the activity of T-reg cells and Clostridia species, mainly C. leptum and C. caccoides, also possess T-reg inducing activity via FoxP3 Helio T-regs and thus protect gut barrier (Lawley & Walker, 2013). The regulation of mucosal immunity is made by Faecalibacterium prausnitzii, and Roseburia species, by increasing Anti-inflammatory IL-10 and reducing Pro-inflammatory Cytokines (IL-12, IFN-γ). The F. prausnitzii also indulge in mucus production, which acts as a barrier for pathogens entry (Wrzosek, Miquel, & Noordine, 2013). The cross talk between host-microbe leading to immune transition is done by B. fragilis, which produces a multi-capsular polysaccharide-A capable of mediating gut - microbiota colonization. Antigen-presentation and immune stimulation is carried out by Akkermansia muciniphila. It produces Propionate, which acts via the Gpr43 receptor and signals the host for activating the genes involved in Ag-presentation (Derrien et al., 2011). Overall, the gut microbiota resists colonisation by pathogens by direct means of competition for niche and nutrition and by indirect means of exploiting host defence mechanism of mediating the activation of neutrophils and secretion of proteases.
Degrading ability
In the distal part of the colon, dietary protein sourced amino acids tyrosine and phenylalanine are converted into -cresol and tryptophan into indole and indole 3-acetic acid. -cresol (a methyl phenol) and indole are partly detoxified by the host through sulfation in the colon mucosa and liver and excreted in the urine. Intestinal mucosa plays a crucial role in detoxification through metabolic processes such as sufation methylation and glucuronidation. Gut microbiota produces a wide range of enzymes such as β-glucosidase, β-glucuronidase, α-rhamnosidase, sulfatase, and esterases, which can hydrolyse glycosides, glucuronides, sulfates, amides and esters, respectively. They also catalyse aromatic ring cleavage, reduction (by reductases and hydrogenases), decarboxylation, demethylation, isomerisation, and dihydroxylation, contributing towards the degradation of complex metabolites.
Secondary metabolites like polyphenols which are abundantly found in plant and plant derived products, usually remain inactive in the diet. Gut microbiota brings about biotransformation into active compounds by removal of the sugar moiety. Structural specificity of polyphenol and individual richness of microbiota determines the level of biotransformation that occur in the intestine (Jandhyala, 2015). In another study (Xu, 2003), degradation of xylene, pectin, arabinose containing indigestible polysaccharides was demonstrated by Bacteroides thetaiotaomicron strain (ATCC29148). This strain has 226 glycoside hydrolases and 15 polysaccharide lyases. In situations when the dietary polysaccharides become low in the host, Bacteroides thetaiotaomicron has the ability to convert these indigestible polysaccharides into digestible one and provides stability to gut ecosystem. Recent studies have shown that increased levels of p-cresol can reduce the capacity of the liver to metabolize acetaminophen due to competitive inhibition of hepatic sulfotransferases. Digoxin, a cardiac glycoside, has been found to upregulate a cytochrome containing operon in Eggerthella lenta (Actinobacteria phyla), resulting in the inactivation of digoxin (Saha, Butler, Neu, & Lindenbaum, 1983).
Fermentation and energy production
The organism of the genus Veilonella produces propionate by fermenting carbohydrates. This propionate enhances insulin sensitivity, Anti-inflammation properties, glucose and energy homeostasis in the host. Methanobrevibacter smithii is a hydrogenotroph that plays an important role in converting the end product of bacterial fermentation (H2) into Methane and thereby increases the fermentation rate. Another important aspect revealed by the experiment with Germ-free mice and healthy normal mice is that gut microbe is essential to ferment unabsorbed starch into SCFA. These SCFA are used by the host as an energy substrate, which contributes to 70% of ATP production in the colon (Donohoe et al., 2011).
Commensalism
Studies have proved that commensalism does occur among microbial species in gut. Organisms which lack “Sialidases” such as Salmonella typhimurium and Clostridium difficle harbour a non-cluster committed for Sialic acid metabolism from other species possessing GH33 sialidases encoding genes (Juge, Tailford, & Owen, 2016).
Disease prevention
Microbes play a major role in reducing disease symptoms and causes. The risk of oxalate stones in the kidney is reduced by the presence of microbes such as Oxalobacter formigenes, Lactobacillus.spp and Bifidobacterium.spp, because these use the end product of carbohydrate metabolism (Oxalate) and thus reducing its conversion to oxalate stones (Magwira et al., 2012).
Lactobacillus strains - L. rhamnosus strain GG, L. acidophilus, L. carei strain GG decrease the effectiveness of diarrhoea caused by acute rotavirus in children and premature infants. L. rhamnosus also show efficacy in treating intestinal C. difficle infections. Presence of the genera - Lachnospira, Veillonella, Faecalibacterium and Rothia in gut healthy individuals prevent respiratory diseases like Asthma (Nylund et al., 2015). Gut bacteria - Bacteroides fragilis, Eubacterium lentum, Enterococcus faecium, Enterobacter agglomerans produce Vitamin K12, which plays a vital role in reducing cardiovascular disorders and coronary diseases. Lactobacillus rhamnosus aids in the reduction of plasma cholesterol, white adipose tissue and triacyl glycerides, which in high levels lead to heart diseases. Even obesity-related disorders are targeted by A. muciniphila by restoring metabolomic endotoxemia induced inflammation and preventing high fat diet induced obesity (Everard et al., 2013).
DNA repair
Microbes are also involved in the indispensable role of DNA synthesis. Bacteria from the genera- Bifidobacterium are the main producers of folate– a vitamin involved in DNA synthesis and repair. It has been demonstrated that Lactobacillus rhamnosus promotes cell renewal and wound healing properties (Swanson et al., 2011). Thus, gut microbes have a promising role in slowing down the ageing process in individuals.
Microbial dysbiosis in Autism spectrum disorder (ASD)
The brain-intestinal axis concept demonstrates the interaction between the brain and the diverse gut microbial population. The gut microbiome in an individual influence the total metabolomic profile and thus has an impact on their overall health (Mohamadkhani, 2018). Intestinal dysbiosis remains a risk factor for several neuron-developmental disorders and physiological diseases such as ASD. Recent findings show that ASD symptoms in affected individuals are linked with intestinal microbiota, which is involved in metabolic pathways and immune regulation (Strati et al., 2017).
Gastro-Intestinal (GI) symptoms, including constipation, diarrhoea which is caused due to the presence of pathogenic microbes in gut, are correlated with behavioural aspects such as anxiety, self-injury and aggression. Bacterial genus such as Prevotella, Coproccus, Veillonellaceae is at a lower level in ASD affected individuals. Even short chain fatty acids (SCFA) synthesizing bacteria, including Faecalibacterium, Roseburia intestinalis and Roseburia faeces are also detected at the lowest levels (Angelis, Francavilla, Piccolo, Giacomo, & Gobbetti, 2015). Also, the beneficial genera Bifidobacterium spp were found to be at a reduced state in children with ASD.
In contrast, there occurred an increase in the levels of the bacterial genera such as Lactobacillus, Clostridium, Bacteroidetes, Desulfovibrio, Sarcina and Caloramator, which had a great connection with autism severity (Kang et al., 2018). The pathogenic microorganism like Sutterella, Enterococcus, Streptococcus thermophilus and toxins (3-(3-hydroxy-phenyl)-3-hydroxy propionic acid (HPHPA), an abnormal phenylalanine metabolite, p-cresol, Indole derivatives) producing organisms including Clostridium difficle, Clostridium histolyticum cluster I & II also prevails in the gut of ASD affected individuals. Gut microbiome enriched with Clostridia, Bacteroidetes and Desulfovibrio produce propionic acid as a resultant metabolite, which is perceived as one of the reasons for autistic behaviours and compromised social manners (Thomas et al., 2012). It was also found that the bacterial genus Sutterella spp. and Ruminococcus torques were highly prevalent in stools of autistic affected individuals. However, the roles of these organisms are not very clear (Wang et al., 2013).
Comparison between neurotypically developed children and those with Autism spectrum disorder revealed that ASD affected individuals had a greater abundance of Bacteroidetes, Parabacteroides, Clostridium, Faecalibacterium and Phascolarctobacterium in their intestinal flora. On the other hand, Coprococcus, Bacteroides and Bifidobacterium were less prominent (Ho et al., 2020).
Clinical studies reveal that the genus Alistipes spp was predominant in individuals affected by depression disorder. Overexpression of Alistipes is linked with other disorders such as Chronic fatigue syndrome and Irritable bowel syndrome (IBS). An in-depth analysis of the intestinal microbiota of affected individuals has also led to the identification of 50 fungal taxa. Among these, Aspergillus, Candida, Penicillium and Malassezia seems to be abundant (Strati et al., 2017).
Microbial metabolites linked with ASD
Studies have shown the elevated presence of p-cresol and sulphate conjugate derivative of p-cresol in autistic affected individuals. Cresol contributes to worsening the severity of autism because it has a deleterious effect on the colon epithelial cells through DNA damage (Andriamihaja et al., 2015). Biochemically, p-Cresol and HPHPA specifically interfere with Dopamine Beta-Hydroxylase, a dopamine converting enzyme. This enzyme is responsible for the conversion of dopamine into norepinephrine, a neurotransmitter. Norepinephrine is a neurochemical associated with vigilant concentration, while dopamine is associated with alertness. When excess dopamine accumulates in the brain, it has negative effects on brain function, such as neurodegeneration associated with oxidative stress.
The other metabolites of intestinal microbiota in ASD are altered SCFA, phenolic derivatives, volatile organic compounds (VOC) and free amino acids (FAA), which have a higher impact on the behavioural pattern in ASD individuals (Angelis et al., 2013). Low level of needed SCFA in ASD affected individuals occurs due to the lower presence of beneficial gut microbes or increased gut permeability. The SCFA has a major role in the regulation and development of neurotransmitters in individuals during their life. It was witnessed that neurotransmitter - Glutamate concentration was higher whereas neurotransmitter - Gamma-aminobutyrate (GABA) was lower in ASD affected individuals (Angelis et al., 2013). Also, the presence of Candida albicans in ASD affected individuals involves the production of hypha-associated cytolytic peptide toxin - Candidalysin and neurotoxin acetaldehyde that induces autistic behaviours like sleep disturbances, sensory issues, hyperactivity, picky eating and stimming (Iovene et al., 2017). The importance of gut microbiome in the development and function of adult brain microglia was demonstrated by Erny and colleagues in a mouse model. The microglia derived from germ-free mice exhibited modified neuro-immunomodulatory factors and transcriptomes, which regulates the cell activation genes (MapK8, Fcgr2β) and type I IFN receptor signalling genes (Jak3, Stat1) when compared with microglia isolated from conventionally colonized mice. Through this study, it is evident that gut microbiota is essential for effective neural functionality in individuals (Erny & Angelis, 2015). More recently, Kang et al. have noted isopropanol to be the most common and recurrent metabolite in ASD patients (Kang et al., 2018). Isopropanol is a neurotoxic solvent that causes GI complications by degrading the gastrointestinal mucosal surface. It also affects the central nervous system and leads to respiratory depression. An animal study has noted Propionic acid (PPA) as a metabolite produced by harmful Clostridia and Desulfovibrio species to be the cause for ASD-like symptoms in mice (Thomas et al., 2012).
Leaky gut theory
Leaky gut is the condition wherein gut barrier integrity gets disturbed, leading to inflammation of gut due to intestinal dysbiosis (Figure 4 ). Due to such leak in gut epithelial lining, bacterial metabolites and toxins get into the systemic circulation. The bacterial lipopolysaccharides (LPS) entering the bloodstream evokes an abrupt immune response to produce increased pro-inflammatory cytokines, which causes inflammation. Thus, disruption in gut barrier due to microbial dysbiosis may allow translocation of bacteria and their antigens, toxins and metabolites. Bacterial antigen lipopolysaccharides, through pro-inflammatory cytokines, can impair neurodevelopment when it happens early in life. Studies conducted among 103 autistic children revealed leaky gut was prominent in 61 children (Kushak et al., 2016). It was evident from the study by Kelly et al. that the presence of the pathogenic Akkermansia muciniphila, a mucin degrading bacteria, serves as the causative factor of leaky gut in ASD affected individuals (Kelly, Minuto, Cryan, Clarke, & Dinan, 2017). This marks the evidence for the occurrence of leaky gut in individuals affected with ASD.
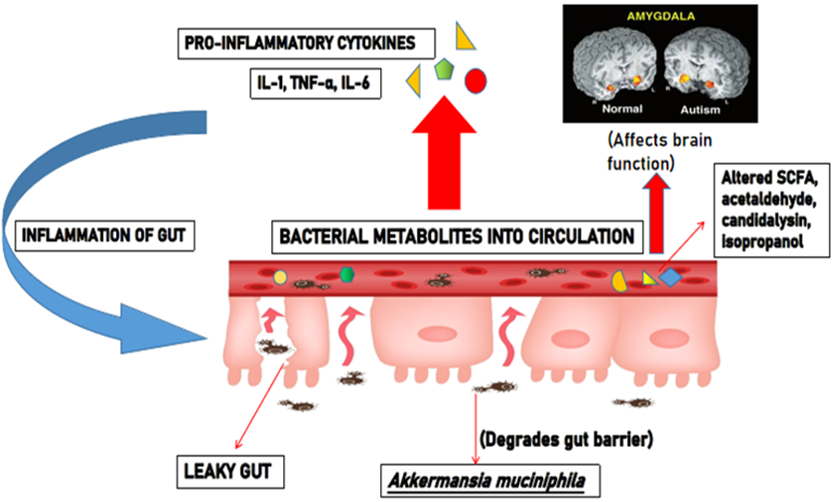
In spite of all the studies and experiments emphasizing the interconnection between gut microbiome and ASD, there are also studies that do not report any relationship between gut microbiota and ASD (Gondalia et al., 2012). Therefore, intense assessment and understanding of the role of intestinal flora in the genesis and development of ASD are much recommended soon.
Role of Microbiota transfer therapy (MTT)
Microbiota transfer therapy (MTT) is the process of transferring beneficial bacteria and other microbes from a healthy individual into the gastrointestinal tract (GI tract) of a recipient. The clinical evidence for microbiota dysbiosis-host interrelation associated with various disorders has made MTT a breakthrough intervention and has gained a prominent role in clinical medicine during recent years. (Surawicz et al., 2013)
MTT techniques
Gut microbial dysbiosis is a condition related to the pathogenesis of intestinal dysbiosis. Studies state that reconstructing the gut microbiota in affected individuals aids in recovery. The different MTT strategies which restore the gut microbiome are,
-
Probiotics administration
-
Prebiotic and Synbiotics
-
Phage therapy
-
Bacterial consortium transplantation
-
Faecal microbiota transfer therapy (Zhang et al., 2018)
Probiotic administration
Probiotics are described as "live microorganisms" that, when provided inadequate amount provides significant health benefits to the host. It provides dual results, as it prevents the onset of dysbiosis and also aids in re-balancing an ongoing condition of dysbiosis (Surawicz et al., 2013).
It is mandatory that the administering probiotic strain should be a normal component of gut microbiota and should be under the "Generally regarded as safe" (GRAS) group. The commonly used probiotic strains in treating gut dysbiosis include lactic acid bacteria, Bifidobacteria, Enterococci, Bacillus spp, and Saccharomyces boulardii (Tian et al., 2016).
There are a few studies based on this method which have been discussed below,
A study by (Grossi, Melli, Dunca, & Terruzzi, 2016) involved the use of "VSL#3", multi-strain probiotics, on a 12-year-old boy diagnosed with ASD. The VSL#3 probiotic mixture had 9 x 1010 cfu/g of Bifidobacterium breve, Bifidobacterium longum, Bifidobacterium infantis and 8 x 1010 cfu/g of Lactobacillus acidophilus, Lactobacillus Plantarum, Lactobacillus paracasei, Lactobacillus bulgaricus, Lactobacillus delbrueckii subspp. The results obtained indicated a reduction in the severity of abdominal symptoms. Autism Diagnostic Observation Schedule (ADOS) score got altered from 20 to 18 post treatment which implied the improvement in autistic core symptoms.
A prospective, open-label was done on 30 autistic children from 5-9 years of age (Shaaban et al., 2018). These children were supplemented with the probiotic formula consisting of 100 x 106 cfu of Lactobacillus acidophilus, Lactobacillus rhamnosus and Bifidobacteria longum strains for three months. The result obtained through Autism Treatment Evaluation Checklist (ATEC) and the six-item GI Severity Index (6-GSI) test showed significant improvement in both gastrointestinal and autism core symptoms.
The efficacy of probiotics as a treatment option to suppress GI symptoms in ASD affected individuals was demonstrated through a randomized controlled study by (Santocchi & Guiducci, 2020). The study involved 85 participants diagnosed with ASD, who were randomly given probiotic or placebo mixture for six months. A patented probiotic mixture "De Simone Formulation" (DSF) containing 450 billion of eight strains (Streptococcus thermophilus, Bifidobacterium breve, Bifidobacterium longum, Bifidobacterium infantis, Lactobacillus acidophilus, Lactobacillus Plantarum, Lactobacillus para-casei, Lactobacillus delbrueckii subsp. Bulgaricus) were given to the participants.
At the end, the probiotic treated subgroups showed a significant decline of 0.81 in ADOS scores and 1.14 in social-affect ADOS CSS scores. Improvements in GI symptoms, adaptive functioning and sensory profiles were also evident.
Prebiotics and synbiotics
Prebiotics are considered specific substances, when given in adequate amounts, that produce significant effects on the host by stimulating the growth and metabolic activity of beneficial gut microbes (Johnsen et al., 2018).
The most commonly used prebiotic supplements include fructo-oligosaccharide supplements, galacto-oligosaccharides, inulin, lactulose and breast milk oligosaccharides. The synbiotic strategy refers to the combination of probiotics and prebiotics in synergism to restore the growth of beneficial intestinal microbes (Luo, Zhang, Shi, Fan, & Ji, 2012).
The effect of prebiotics was evaluated in 30 autistic children for 6 weeks in a study by (Grimaldi et al., 2018). Their study indicated that prebiotic intervention "Bumino ® galactooligosaccharide” (B-GOS ®) ameliorated Fecalibacterium prausnitzii and Bacteroides spp. levels in the study individuals and also improved their anti-social behaviour.
Although these studies indicate the role of probiotics as a possible mode of treatment, long term, systematic follow-up studies are needed to assess if normal microbiota has been restored in the treated individuals.
Phage therapy
This therapy involves the use of "Bacteriophages", which infect bacteria. Genetically modified phages are used as gene carriers that exhibit anti-microbial activity and alters the gut microbial community back to a healthy state. Phage therapy protocols were not designed based on the "International community for medical research" criteria and hence are yet to be approved by FDA (Surawicz et al., 2013).
Bacterial consortium transplantation
Bacterial consortium transplantation (BCT) focuses on targeted restoration of intestinal microbiota. It involves the purification and culture of a specific faecal microbial population for treatment. Studies depict that the effects of BCT are effective and comparable to those of faecal microbial transplantation (FMT) in modulating intestinal dysbiosis.
(Li et al., 2015) demonstrated that Bacterial Consortium Transplantation (BCT) would be an effective bio- therapeutic strategy in restoring the intestinal ecosystem. They conducted the study using a mice model treated with "Ceftriaxone" intra-gastrically for 7 days which cleared out all the microbial population in gut environment. Following this, BCT was performed for 3 consecutive days to rebuild the gut microbiome. The BCT mixture was prepared using 11 species belonging to the phyla Firmicutes, Proteobacteria, Actinobacteria, Bacteroidetes and Fuscobacteria. These BCT strains were cultured from the faecal content of healthy mice using selective media. The results were analyzed using Denaturing gradient gel electrophoresis (DGGE) and Quantitative Polymerase Chain Reaction (qPCR), which indicated that the microbial community in treated groups had established back to the level of donor/control mice within three weeks. It also normalized the secretory levels of MUC2, SIgA and Defensin in the intestine.
Faecal microbiota transfer therapy (FMT)
The most common and widely used MTT technique is "Faecal microbiota transfer" (FMT), which is popularly called "Poop pills". The incorporated microbial species start constructing the normal healthy gut microbiota, which eventually disrupts the pathogenic microbial community and prevents them from recurring. Thus, FMT is used to lower the severity of diseases associated with microbial dysbiosis (Surawicz et al., 2013). FMT has been approved as a standard therapy module to treat Clostridium difficle infection. It is also used to treat refractory ulcerative colitis, Crohn's disease, constipation, irritable bowel syndrome and ASD. FMT is considered to be the very effective and promising strategy of MTT (Johnsen et al., 2018; Tian et al., 2016)
History of FMT
Current findings declare that the medicine book “Zhou Hou Bei Ji Fang” written by “Hong Ze” in China depicts the first record of using human faeces to treat human diseases. The book described the use of FMT therapy in curing serious disorders in the 4th century which includes, food poisoning, “Wen Bing” (febrile disease), “Shang Han” (typhoid fever) and refractory conditions (Zhang, Amateau, Khashab, & Okolo, 2012). Further, in 1958, Eiseman et al. Proved the effective role of faecal enemas in treating severe ‘Pseudomembranous colitis’ in affected individuals (Surawicz et al., 2013). Recently in 2013, FMT therapy was standardized for its treatment guidelines on recurrent clostridium difficle infection. As FMT was accepted as an effective treatment for several diseases, it evolved into a new strategy called 'Selective microbiota transplantation' (SMT). SMT based studies focus on the use of specific microbiota in different organs other than gut, such as the vagina, sinus, urinary tract and skin.
FMT Procedure
The main goal of FMT is to restore the normal microbial community in a dysbiotic gut. To achieve this, a strict and standard protocol is followed in the FMT procedure. The FMT procedure is comprised of several steps, which includes: Selection and screening of donor, stool analysis, screening of recipient, FMT preparation and administration (Vindigni & Surawicz, 2017)
Selection and screening of donor
The donor screening protocol is mandatory to avoid the transmission of an infectious organism from donor to recipient. The donor may be related or unrelated to the recipient. The clinical history of the donor is analysed before the start of the treatment. The donors who had exposure to antibiotics in the prior three months, autoimmune or allergy history, gastrointestinal malignancy, drugs and body piercing in the prior six months are strictly excluded.
Stool analysis
The donor stool sample possesses the presence of Bacteria such as Clostridium difficle, Campylobacter, Helicobacter pylori, Salmonella, Shigella, and Yersinia spp., Viruses such as Rotavirus, Norovirus and Parasites such as Cryptosporidium, Cyclospora and Isospora are not considered to be ideal for donor samples.
Screening of recipient
There is no particular screening protocol for the recipients, although the immunocompromised status of the recipient is analysed, as it may increase the risk of adverse events. Tests for the presence of viral hepatitis, human immunodeficiency virus and syphilis are done in certain cases.
FMT preparation
The FMT preparation varies based on the disease treated, research practitioner and institutions. The basic process involves:
Collection of stool → Screening of stool → Mixing of stool with bacteriostatic liquid → Filtering out the particulate matter → Faecal extract → Loading into syringe → Administration to affected individuals.
The donor sample is obtained via three different methods, which could be either a Stool sample directly collected from a donor or frozen stool sample obtained from a stool bank or frozen, pre-prepared encapsulated stool. Recently, it was suggested by researchers that the preparation of faecal microbiota can be classified as (a) Rough filtration (RF), (b) Filtration plus centrifugation (FPC), (c) Microfiltration plus centrifugation (MPC) (Kang et al., 2017)
Administration
The administration route for microbial transplantation in recipients is done by three versatile methods (Figure 5) :(a) Upper-gut, (b) Mid-gut and (c) Lower-gut (Peng et al., 2016). Usually, the selected microbiota is converted into a powder or suspension form and given orally as a "Poop pill". It is infused via mid-gut through various techniques, which includes, Nasojejunal tube, Transendoscopic enteral tubing (TET) and Percutaneous endoscopic gastro-jejunostomy (PEG-J) (Zhang et al., 2012). Lower-gut infusion involves different Colonoscopy, Enema, Distal ileum stoma and Colonic TET (Peng et al., 2016). Figure 6 lists the various advantages and disadvantages associated with different routes of administration in FMT (Vindigni & Surawicz, 2016).
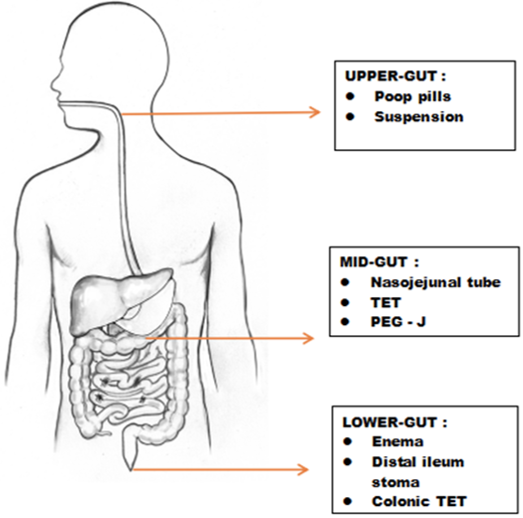
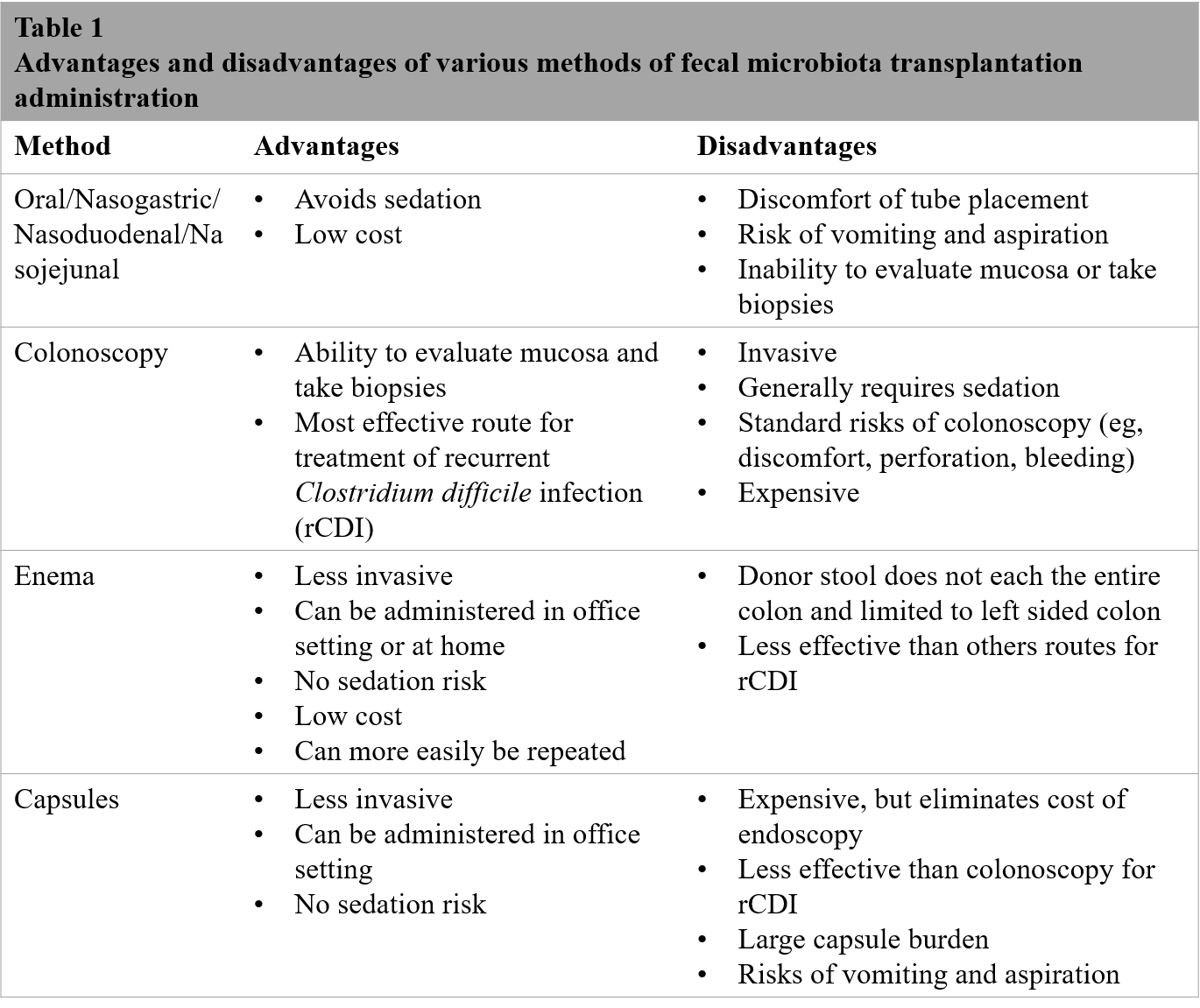
Adverse effects of FMT
Faecal microbiota transplantation or intestinal microbiota transplantation has shown effective results in therapeutics, despite the fact that it is also associated with some adverse effects (Wang et al., 2016). The minor side effects include nausea/vomiting, abdominal pain, bloating, flatulence, diarrhoea and low-grade fever. The severe adverse effects are sedation related aspiration, endoscopy related bleeding or perforation, infection or sepsis, inflammatory bowel disease and risk of chronic disease due to the change in gut microbiome (Vindigni et al., 2017).
Role of microbiota transfer therapy in Autism spectrum disorder (ASD)
Intestinal dysbiosis serves as the major factor for autism spectrum disorder, through the "gut-brain axis", wherein, the microbial metabolites have a greater impact on the brain's functionality and neural development in an individual. Many studies have discussed the efficacy of MTT in restoring the dysbiotic environment, which eventually decreases the severity of ASD in affected individuals. The available clinical studies that have emphasized the significant role of MTT in ASD treatment have been discussed below:
(Kang et al., 2017) performed an open-label study involving MTT wherein they discussed improvements in Autism symptoms post gut microbiota transfer therapy (MTT) in ASD. Their study group involved 18 ASD diagnosed children (7-16 years) for 18 weeks which included a 10-week FMT treatment followed by an 8-week observation period post-MTT. As a control group, 20 age- and gender-matched neurotypical children without GI disorders were recruited. Neurotypical children were also monitored for 18 weeks but were not treated. The experiment protocol involved four major steps, (i) oral vancomycin, (ii) Moviprep (Bowel cleansing), (iii) SHGM (iv) Prilosec.
The treatment for the ASD group began with providing 14 days of oral vancomycin to subside pathogenic bacteria. On day 15, "Moviprep", a drink, was administered to affected individuals to cleanse the vancomycin residues retained in the bowel. On day 16, oral/faecal administration of "Standard human gut microbiota" (SHGM) with 2.5 x1012 cells/day concentration was started and followed by a lower dose of (2.5x104 cells/day) was given until the end of the treatment schedule. Additionally, Prilosec (acid pump inhibitor) was administered daily to affected individuals to suppress stomach acidity and increase the SHGM survival rate starting from day 12 of the treatment schedule. The SHGM obtained from the donor was screened for potential pathogens such as Clostridium difficle, Campylobacter, Salmonella, toxin producing Escherichia coli, Vibrio, Yersisnia, Enterococcus, Giardia, and Cylclospora species, potential parasites and potential viral pathogens before administering. Assessment of gastrointestinal symptoms by "Daily stool records" (DSR) and (GSRS), autism and related symptoms by structured interview, NextGen sequencing and virome population analysis were performed pre and post the MTT treatment.
The results obtained from the study indicated that more than 85% of the affected individuals achieved a 50% reduction in average GSRS scores. The average developmental age of affected individuals increased by 1.4 years among all sub-domain areas. It was detected that 95.64% of phage populations were unknown and the rest belonged to the Caudovirales order. Beneficial microbes such as Bifidobacterium, Prevotella and Desulfovibrio levels were increased post treatment. Correlation between microbiome and ASD was evident as the treated patient showed a successful shift of ASD bacterial community towards healthy controls.
It was concluded that the ASD affected individuals treated by MTT had gained partial engraftment, shift towards healthy gut microbiota and eventually ended up exhibiting significant improvements related to both GI and ASD symptoms.
A follow-up study to this (Kang et al., 2017) was done by the same group on the 18 ASD affected individuals two years after the initial MTT treatment (Kang et al., 2019). They reported a change in GI and autism related symptoms.
A 58% reduction in Gastrointestinal symptom rating scale (GSRS), 26% reduction in Daily stool record (DSR) were noted when compared with baseline results obtained in the study two years earlier. The severity of ASD was 47% lower than the baseline range on the Childhood autism rating scale (CARS). The Parent rated social responsiveness scale (SRS) revealed a percentile drop in ASD severity, while the Parent global impressions-III (PGI-III) scores remained the same. The study proves that the gastrointestinal and ASD symptoms were interconnected, as the CARS, ABC and SRS scores were positively correlated with GSRS scores in the follow up test. This study implied the long-term potential effect of MTT intervention in reducing the severity of GI and ASD behavioural symptoms.
Further to their earlier studies, analysis of complete metabolite profile of faecal and plasma samples of 18 ASD affected children post MTT trial and control group were performed by the same group (Kang et al., 2020). The same MTT protocol used in the (Kang et al., 2017) study was followed one more time by studying the plasma and faecal metabolite profile.
The result indicated the decreased range of medium-chain fatty acids (Caprylate and Heptanoate) after MTT treatment and a significant increase in the levels of nicotinamide riboside, IMP, methyl succinate compared to the baseline range before treatment. p-cresol sulfate, whose level was relatively higher in the ASD group at baseline, showed a decrease in levels after the MTT treatment, similar to the levels in typically developing (TD) controls. The levels of p-Cresol sulfate were inversely correlated with Desulfovibrio, suggesting a potential role of Desulfovibrio on p-cresol sulfate modulation. The alterations in the metabolite profiles and their role in autism spectrum disorder was apparent from this study. Outcome of the study has been depicted in Figure 7. (Kang et al., 2020).
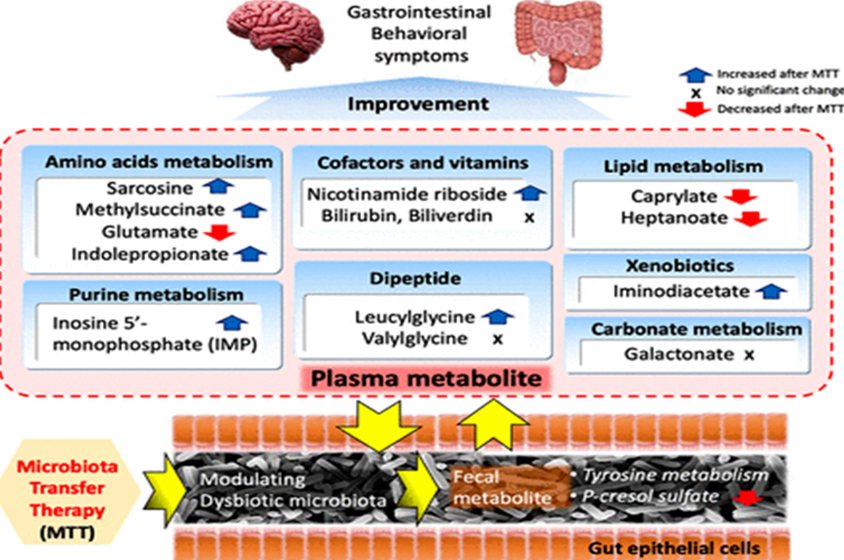
In another study, the efficacy of oral and enema FMT combination in treating the late onset of ASD was checked in nine differently aged children subjects. The protocol involved post oral vancomycin treatment for 7-10 days, followed by the ingestion of gelatin capsules containing > 6ml of concentrated faecal organism extracted from healthy donor stool. It was finally completed with an enema of faecal slurry (50-500ml). Test individuals’ faecal samples pre and post FMT and post vancomycin treatment were collected and 16SrRNA sequence analysis of individual isolates were performed. Results obtained indicated microbial community alterations consisting of Bacteroides, Barnesville, Sutterella and Clostridales post FMT treatment. They observed a marked improvement in ASD symptoms in younger subjects on a long-lasting basis through their study.
Animal based study was also performed to check the efficacy of FMT. Potential effects of FMT on autistic like behaviour was tested in Fmr1 KO mice (Fmr1 gene knock-out mice). Fmr1 KO mice exhibit retarded growth and development as they lack FMRP (Fragile X mental retardation protein), essential for synaptic plasticity and cognitive development. After the completion of the FMT protocol, expression of MUC2 (Mucin 2, Oligomeric Mucus/Gel-Forming) and goblet cells in gut were analysed and compared to the results obtained before treatment. Results obtained in this study indicated the normalization of MUC2 expression and autistic-like behaviour (Memory deficits and social withdrawal) in treated mice. It was also evident that the Akkermansia muciniphilla got elevated, whereas TNF-α and Iba1 levels decreased (higher levels of these lead to necrosis of cells). This animal study suggests that FMT could be a beneficial method in treating autism spectrum disorder.
Other methods of treatment tried in ASD
Hyperbaric Oxygen Therapy (HBOT)
Many reviews have suggest that Hyperbaric Oxygen Therapy (HBOT) for autistic affected individuals potentially increase cerebral perfusion and revert the mitochondrial dysfunction. In the first controlled study on the role of HBOT on ASD, (Rossignol et al., 2012) included 62 autistic children and provided them with 40 hourly treatments of HBOT. Two groups- the Treatment group and Control group were segregated. The treatment group received 24% Oxygen at 1.3 atm and the control group received 21% Oxygen at 1.03 atm. The results obtained through the "Clinical global impression scale" (CGI) indicated that the treatment group were rated under the "much improved" range when compared to the control group. The "Aberrant behavior checklist”(ABC) stated that significant improvements in the total score of stereotypy, hyperactivity, irritability and speech were observed in the treatment group. In the Autism treatment evaluation checklist (ATEC), sensory/cognitive awareness, social interaction and overall functioning levels were improved in participants who underwent HBOT treatment. Although the study gave encouraging findings, it was not designed to measure the long-term outcomes of hyperbaric treatment in children with autism. Hence additional studies were required to determine if the significant improvements observed in this study lasted beyond the study period.
In a subsequent study, 1.5 atm/100% oxygen was delivered in 80 HBOT sessions for 20 weeks in 10 children with ASD. Plasma cytokine levels, including some associated with inflammation, were measured before and after the sessions. Behavioural improvements were observed in these children, but the study did not report any significant changes in cytokines during the study as the children did not have any abnormal cytokine levels at the beginning of the study. (Bent, Bertoglio, Ashwood, Nemeth, & Hendren, 2012)
These studies have focussed primarily on measuring inflammation and oxidative stress changes before and after HBOT in children with autism.
In a clinical trial from Thailand (Sampanthavivat, Singkhwa, Chaiyakul, Karoonyawanich, & Ajpru, 2012), 60 children aged 3 to 9 diagnosed with ASD received 20 1-hour sessions of either HBOT (1.5 ATA and 100% oxygen) or a placebo treatment (1.15 ATA and 21% oxygen) on consecutive weekdays throughout a 10-week period. Following HBOT treatment, The participants’ amount of social interaction and communication, behavioural problems, communication and linguistic abilities, and cognitive function were rated by both parents and trained clinicians using the Autism Treatment Evaluation Checklist (ATEC) following HBOT. There were no significant differences found in either the parent- or clinician-completed ATEC findings when comparing children who had received HBOT with those who had received placebo treatment. The study concluded that there was no overall benefit from HBOT (Sampanthavivat et al., 2012).
Currently, there is insufficient evidence to support the use of HBOT to treat children with ASD due to conflicting findings from reported studies. More structured research with long term follow-ups may reveal the beneficial aspects of this therapy.
Chelation therapy- use of Dimercapto succinic acid (DMSA)
Chelation refers to a medical procedure that uses chemicals to remove heavy metals from the bodies of children with autism. The agents most commonly used are Calcium, Disodium ethylenediamine-tetraacetic acid (Ca-EDTA), Dimercaptosuccinic acid (DMSA) and 2-3-dimercaptopropane-1-sulfonate (DMPS). These chemicals have a high affinity to bind with metals and remove them from the body via urine when the drug is excreted. The administration of metal-binding molecules to treat autism is based on speculation that mercury poisoning (via administration through immunization, mercury-containing dental fillings or environmental exposure) causes autism.
Therefore, the rationale is that removing the toxic metal will cure autism or lead to an amelioration in the symptoms of autism.
A study by (Adams et al., 2009) illustrated the efficacy of chelation therapy using oral Dimercapto succinic acid (DMSA) in children with an autism spectrum disorder.
It was conducted in two phases. In phase-I, 65 children received one dose of DMSA for three days. Out of that, only 49 participants moved to phase-II, wherein they received extra six doses of either DMSA or placebo in a double-blind method. The obtained result suggested that DMSA therapy at the dosage used in this study was safe and effective in removing toxic metals such as lead, bismuth, tin, thallium, mercury, antimony and tungsten, suppressing platelet levels and normalizing glutathione levels in affected individuals with ASD.
This therapy also led to the loss of required potassium and chromium levels; hence supplementation of these minerals was suggested to the participants (Adams et al., 2009).
As a follow-up to their previous study, they determined the behavioural alterations caused due to oral DMSA therapy in ASD affected individuals (Baral et al., 2009). Analysis was made to check the relationship between urinary excretion of toxic metals through oral DMSA and the severity of autism. Tools such as pervasive developmental disorder behaviour inventory (PDD-BI), autism treated evaluation checklist (ATEC), the severity of autism scale (SAS) and autism diagnostic observation schedule (ADOS) were used to assess the autism severity levels in the subjects. It was evident that the DMSA therapy improved the autism severity score in 77% of the participants, provided no change in 12% and led to a worsened result in the rest 11% of participants.
Even when used appropriately in a clinical setting, chelation can have side effects ranging from dizziness and nausea to headache and diarrhoea. When used inappropriately and/or without proper supervision, chelation can have very serious side effects ranging from liver damage, kidney damage and brain damage that can be life-threatening. The risks listed outweigh the benefits derived. Known side effects of these chemicals include Two reported deaths, hypocalcemia and depletion of beneficial metals (zinc, iron). Ten percent of DMSA-treated patients showed evidence of gastrointestinal issues, including elevation in liver enzymes. At this point, there is insufficient scientific evidence to consider chelation therapy as a treatment option for ASD, with their potential for adverse side effects (James et al., 2015).
Future Perspective
The prevalence of autism spectrum disorder is increasing globally, hence diagnosis and treatment interventions have to be developed appropriately to meet the needs. Studies using the preclinical model should be encouraged to understand the epigenetics and other factors involved in ASD and discover effective targets for novel treatment. Early identification of neurobiological mechanisms in ASD will be critical for enhancing the success rate of treatments. Multi-omics approaches can also be utilized to define the roles of specific gut microbes with the context of neural developmental issues. Instead of focussing on the microbial composition of gut, research should shift towards determining the mechanism of the metabolites or end products produced by them. More studies involving gut-microbiota mediated metabolites and immune system related biomarkers would lead to an easy and accessible diagnosis and treatment interface for ASD. In future, it might eventually be helpful to initiate a diagnosis of pre-ASD or prodromal ASD that could be given before a child meets full ASD standards.
Currently, from the available studies, MTT remains to be a potential treatment method for ASD; however, clinical trials with broader, longer observation period after treatment would help deciding the long-term safety and potential advantages. Manufacturing of MTT should be standardized and the development of such products should be coupled with rigorous mechanistic research. Knowledge and standardization of ideal donor selection, the microbial composition of a stool sample, the best route of administration and dosage is mandatory for safety concerns. As there are only limited studies based on MTT intervention for ASD, there is a need of more clinical and well-designed studies that include larger samples to provide robust evidence which supports the effectiveness of this treatment intervention (Kang et al., 2017).
Further exploration on clinical uses of neurotechnology for mental health issues are arising. Brain-computer interface (BCI) and other such methodologies might be valuable in assisting communication and social weakness in ASD affected individuals.
Conclusion
Autism spectrum disorder (ASD) is a neurological developmental disorder which affects the communication and social behaviour of an individual. Gastrointestinal symptoms are one of the major associated comorbidities in ASD due to microbial dysbiosis in the gut flora. As gastrointestinal symptoms prevail in all cases of ASD, it is now evident that gut microbial dysbiosis remains a significant factor for the severity of ASD symptoms. While many ASD affected individuals are provided with behavioural therapies as a treatment modality, there is negligible proof to exhibit their efficacy. The medications used to suppress the gastrointestinal symptoms do impart positive results, but the affected individuals remain susceptible to adverse side effects of the given medications. As the gut–brain axis has a vital role in the prevention and treatment of neuropsychiatric disorders like ASD, treatment based on altering the gut dysbiosis remains effective. One such treatment is MTT (Microbiota transfer therapy), wherein the microbiota from a healthy donor is transplanted to the affected recipient to restore their gut flora. As the success rate of MTT intervention is higher compared to other treatment modalities, FDA granted “Fast track” status to the use of microbiota transplant therapy for ASD in 2019. Hence, it is apparent that MTT intervention potentially manipulates the dysbiotic intestinal microbial communities in recipients and thereby provide promising results in treating the broad-spectrum autism disorder. Despite these evidences, there are only limited available data based on MTT intervention for ASD. There is a need for more structured clinical investigations that include a higher sample size, targeted biomarker analysis, standardized protocol and personalized treatment method to prove its potential usage in ASD treatment.
Conflict of Interest
The authors declare that they have no conflict of interest for this study.
Funding Support
The authors declare that they have no funding support for this study.