Synthesis and Characterization of CSNP-SiO2 nanocomposite by using antibacterial activity
Abstract
Because of its promising biological achievements, the development of nanotechnology, nanoparticle-based products, and their applications has piqued the interest of many researchers. Inorganic nanomaterials, on the other hand, are well known to be effective antimicrobial agents. Metal nanoparticles, such as Silicon dioxide, are particularly important among the various nanoparticles due to their low cost and ease of availability. Chitosan is a biopolymer derived from chitin, a natural polysaccharide that follows cellulose as the second most abundant polysaccharide. The Precipitation Method was used to create a Chitosan Nanoparticle with SiO2 Nanocomposite (CSNP - SiO2 nanocomposite). TGA, XRD, SEM, FT-IR, PSA, UV, and FL were used to characterize the Chitosan Nanoparticle with SiO2 Nanocomposite. The findings showed that bionanocomposites have a greater antibacterial effect due to the combined effect of CS and nanoparticles (NPs). Chitosan/SiO2 nanocomposites were tested for antimicrobial activity against gram-positive and gram-negative microorganisms. The goal was to assess their physical, mechanical, and biological properties, as well as their potential for biomedical applications.
Keywords
Chitosan, chitosan NPs, CSNP - SiO2 nanocomposite, Antibacterial activity, TGA, XRD, SEM
Introduction
Nanobiotechnology has emerged as a fundamental field of current research as a cutting-edge innovation that is interdisciplinary with physics, chemistry, and biology (Kafshgari et al., 2019; Rehan, El-Naggar, Mashaly, & Wilken, 2018). During the last decade, nanomaterials demonstrated significant antimicrobial activity against human, animal, and plant pathogens (Bhat et al., 2020). Researchers refer to this potential as their distinct optical, electronic, or mechanical properties because of quantum and surface boundary effects compared to bulk materials (Luo & Stutzenberger, 2008). Nanocomposite materials have been used in various applications, including wound healing, tissue engineering, thermal therapy, and drug delivery (Shabunin et al., 2019). Chitosan is a biocompatible polymer made up of polysaccharide with antibacterial properties that has been used in wound dressings (Cremar et al., 2018). Chitosan is a biopolymer composed of N-acetylglucosamine and glucosamine. It has many properties, including non-toxicity, biocompatibility, biodegradability, moisture retention, and low cost (Alba et al., 2019; Alshammari, Essawy, El-Naggar, & Sayyah, 2020; Liu et al., 2006). The antimicrobial activity of chitosan polymers and nanoparticles can be attributed primarily to their electric charge and high adsorption ability, as well as chemical reactions that allow them to interact efficiently with the bacterial cell membrane (Preethi et al., 2020; Wang et al., 2020), whereas the inhibitory effect on bacterial growth is dependent on their sizes and shapes, as well as biological properties (Chandrasekaran, Kim, & Chun, 2020; Wang et al., 2020). When chitosan and metal nanoparticles are combined, their antibacterial activity may be significantly inhibited compared to the antibacterial activity of chitosan and metal nanoparticles alone (Figure 1 ). Chitosan has been studied as the primary structural unit of nanomaterials due to its low toxicity, non-immunogenicity, and biodegradability. Li et al. (2010) 13, for example, A chitosan/TiO2 nanocomposite with antibacterial activity against Xanthomonas oryzae PV. oryzae was synthesised and characterised. In this study, we report on the preparation of a silicon dioxide – chitosan nanocomposite by chemical reduction of corresponding metal ions into zero valent nanoparticles in the presence of chitosan. The morphology of these nanocomposites was studied in addition to their optical and other properties. Antibacterial activity was evaluated against five gram-positive (Bacillus subtilis and Staphylococcus aureus) and gram-negative microorganisms (Enterobacter, Escherichia coli and Pseudomonas fluorescens).
Materials and Methods
CDH Chemicals New Delhi supplied the following analytical grade chemicals: silicon dioxide (SiO2), Chitosan, sodium tripolyphosphate (STPP), sodium hydroxide, potassium permanganate, oxalic acid, acetic acid, hydrochloric acid, and sodium hydroxide.
Synthesis of Chitosan nanoparticle
The method of ionic gelation was used to create chitosan nanoparticles from chitosan. To make a chitosan solution, 0.5g of chitosan was dissolved in 5% acetic acid and stirred for 30 minutes. Sodium tripolyphosphate, a cross-linking agent, was added dropwise. A magnetic stirrer was used to mix the mixture at the same time. After stirring at room temperature, the solution was centrifuged for 30 minutes and kept in a hot air oven to obtain chitosan nanoparticle powder. Chitosan nanoparticles were created from the white powder that was obtained.
Synthesis of CSNP-SiO2 nanocomposite
0.5g of Chitosan nanoparticles were mixed with 100 ml of distilled water, and the solution was supplemented with 0.5g of SiO2, as shown in Figure 2. To keep the reaction going, constant stirring was used. The reaction was kept going for about 2-4 hours. After that, it was centrifuged for 30 minutes and dried in a hot air oven at 1000 degrees Fahrenheit. The powder was ground with a mortar and pestle and calcined for 8000 seconds to produce a CSNP-SiO2 nanocomposite.
Antibacterial Property
The agar diffusion method was used to test the antibacterial activity of CSNP-SiO2 nanocomposite against the bacterial strains Staphylococcus aureus, Bacillus subtilus, Enterobacter, Escherichia coli and Pseudomonas fluorescens. Bacterial strains were grown overnight on a rotary shaker in a Nutrient broth medium in a single column system. The same is kept for 24 hours in a sophisticated incubator for incubation. To determine the bacterial effect of the CSNP-SiO2 nanocomposite, a loop of bacterial culture was placed on the Muller Hinton broth medium. After 24 hours, the zones of inhibition were measured under the same conditions.
Results and Discussion
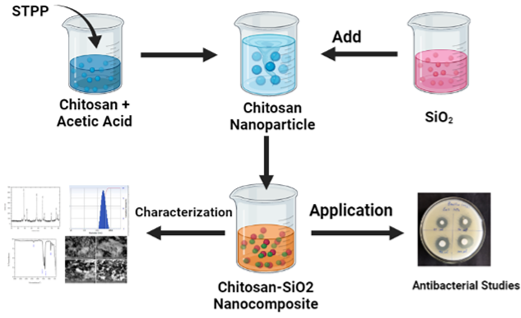
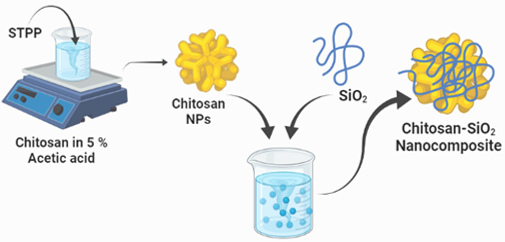
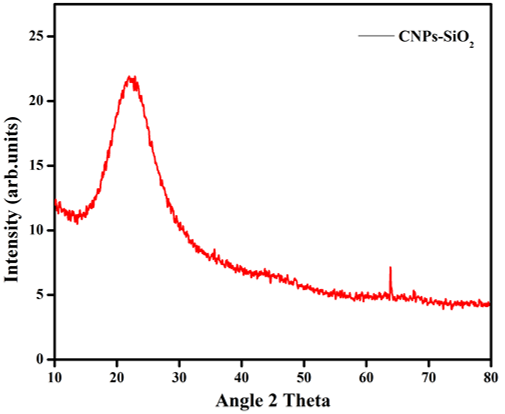
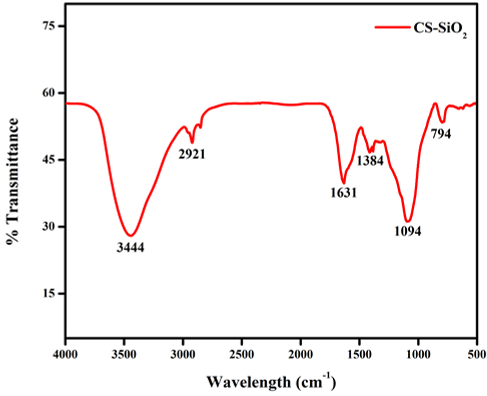
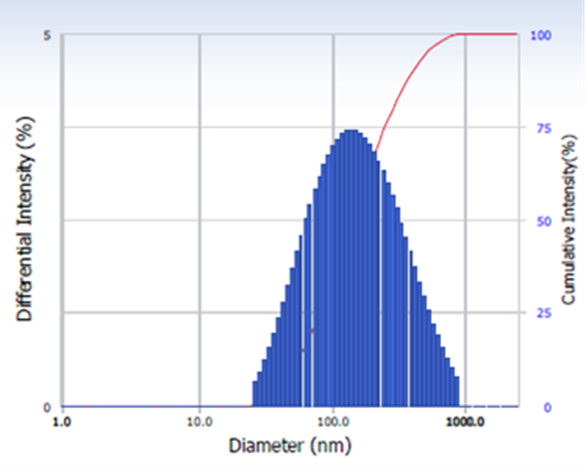
XRD Diffraction
The CSNP-SiO2 XRD pattern (Figure 3) revealed ultrafine powder samples obtained from sol. The result shows an amorphous structure rather than a crystalline structure. The crystalline structure was expected to result from the sample being heat treated at a high calcination temperature (i.e.,50°c).
The diffractogram (Figure 3) was compared to the JCPDS standard powder diffraction card, CSNP-SiO2 file No. 00-001-1262. CSNPs are amorphous with no crystalline peak in the XRD pattern, but when incorporated with SiO2, they exhibited characteristic crystalline peaks at 2 values of 22.91° and 64.95°, corresponding to (hkl) values - (111) and (200) planes of CSNP-SiO2. The XRD analysis confirmed that the particles in the prepared sample are CSNP-SiO2 with a face-centred cubic crystal structure (Al-Sagheer & Muslim, 2010). As the peak intensity of polymer nanocomposite films increases, the amorphous nature of polymer nanocomposite films decreases, while the semi-crystalline nature of polymer nanocomposite films increases (Meftah, Gharibshahi, Soltani, Yunus, & Saion, 2014). The stable structure of the hydrogen bonds between water molecules and the amino groups of chitosan has previously been reported, as the water, molecules are due to the crystalline region of chitosan (Meftah et al., 2014).
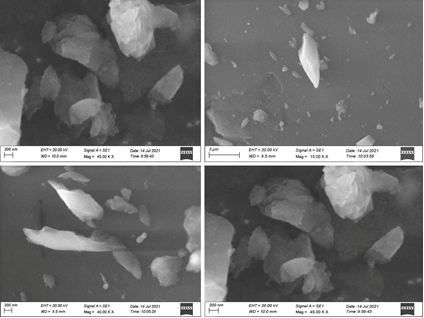
Fourier-transform infrared spectroscopy
The bonding nature of the CSNP-SiO2 nanocomposite was investigated using an infrared analysis tool. Figure 4 depicts the FT-IR spectra of a CSNP-SiO2 nanocomposite. The samples' IR spectrum was examined at wavelengths ranging from 4000 to 400 cm-1. The peak at 3444 cm-1 was more prominent than the others, indicating -NH2 and -OH stretching primary amines. The presence of C–H stretch alkanes was suggested by the peak 2921 cm-1 (Wang et al., 2020). Peak 1631 cm-1 indicates the presence of C=C stretching alkene, while 1094 cm-1 indicates C-O stretching aliphatic ether and 794 cm-1 indicates the presence of C=C bending alkene compound. Previous studies Wang et al. (2020) reported similar results for the formation of chitosan nanoparticles treated TPP. The FTIR spectral absorbance of CSNP-SiO2 nanocomposite shows all of the SiO2 and biopolymer chitosan vibrations. The observed functional groups indicated that chitosan might be responsible for the reduction and formation of SiO2, and the other chitosan functional groups present in CSNP-SiO2 nanocomposite support chitosan coating on synthesized CSNP-SiO2 nanocomposite. The FTIR spectral absorbance of the CSNP-SiO2 nanocomposite shows that all the SiO2 and biopolymer chitosan vibrations are present. The observed functional groups suggested that chitosan may be responsible for SiO2 reduction and formation, and the other chitosan functional groups present in CSNP-SiO2 nanocomposite support chitosan coating on synthesised CSNP-SiO2 nanocomposite (Sorrentino, Gorrasi, & Vittoria, 2007). FT-IR techniques are one of the most effective methods for investigating interactions between a polymer matrix and a CSNP-SiO2 nanocomposite material.
The mechanical properties of the CSNP-SiO2 nanocomposite can be explained using a detailed analysis of FT-IR spectra.
The formation of hydrogen bonds and van der Waals interactions between the phases increased the mechanical parameters of the nanocomposite material (Paluszkiewicz, Stodolak, Hasik, & Blazewicz, 2011).
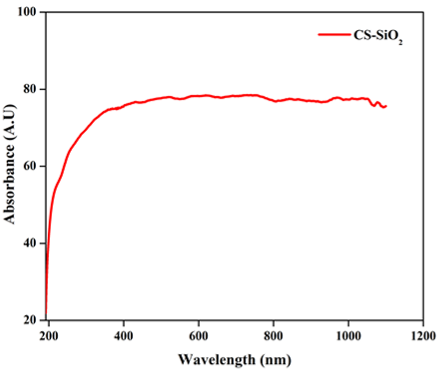
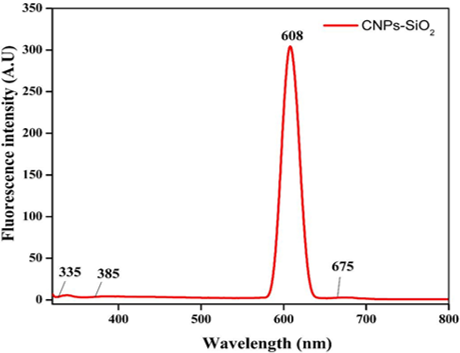
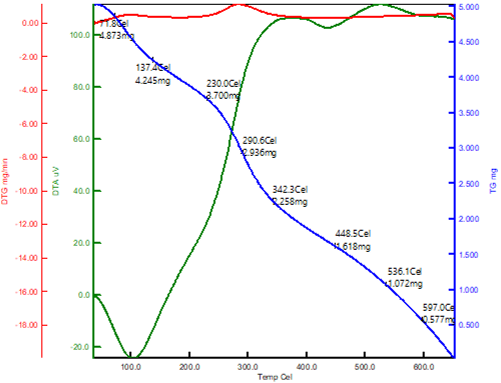
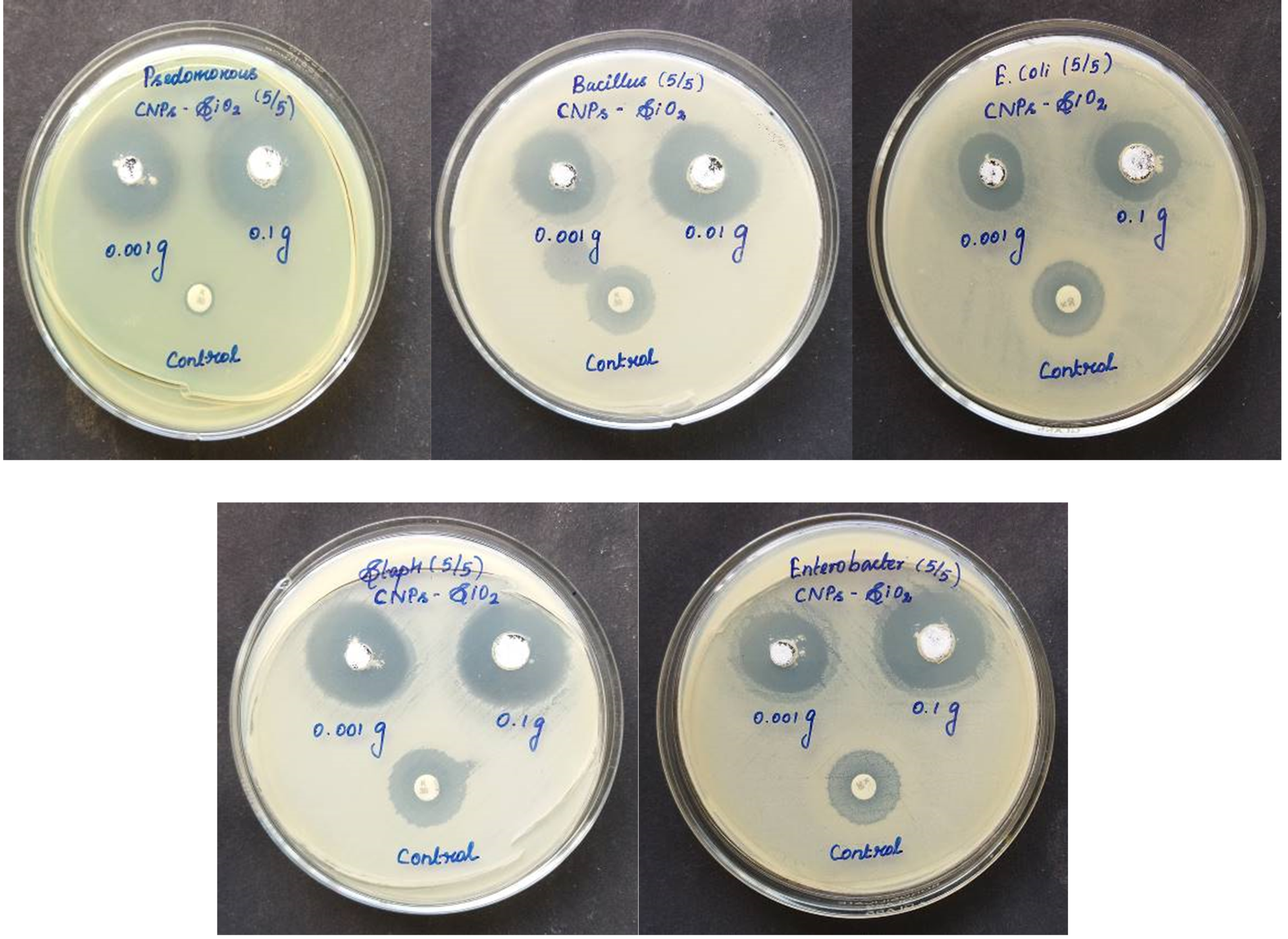
Particle Size Analyzer
The diameter of nanoparticles dispersed in liquid is measured using the DLS, as shown in Figure 5. It also determines particle size and distribution in physiological solutions. DLS can measure the size distribution of small particles in solution or suspension on a scale ranging from submicron to one nanometer (Akbari, Tavandashti, & Zandrahimi, 2011). This method can measure narrow particle size distributions, particularly in the 2–500 nm range (Longo, Carotenuto, Palomba, & Nicola, 2011). Dynamic light scattering is a method that relies on light interaction. The dynamic light scattering (DLS) analysis of the CSNP-SiO2 nanocomposite shows a particle diameter average of 75 nm with a polydispersity index of 0.382.
Scanning Electron Microscopy
As shown (Figure 6 ) in the SEM images, the undefined and whitish SiO2 nanoparticles are dispersed uniformly within the silicone elastomer specimens. The nano-SiO2 concentrations were distributed uniformly throughout the silicone specimens, according to SEM analysis. As the SiO2 nanoparticles loading were increased in all specimens, no aggregates were detected.
The morphology of the CSNP-SiO2 nanocomposite was determined using scanning electron microscopy. Because of the polymeric nature of CS and SiO2 on the polymer matrix, the CSNP-SiO2 has an undefined shape with agglomeration. At (20 and 2lm) magnification. The surface morphology and uniform distribution of CSNP-SiO2 nanocomposite in the polymer nanocomposite are spherical, as shown in Figure 6, as is the shape of encapsulated nanoparticles in the polymer nanocomposite (Figure 6). SEM images can create a histogram with Image J software to determine the nanoparticle's small size and even distribution (Xu, Shi, Yang, & Li, 2016). Scanning electron microscopy studies show that the cross-linked SiO2 materials have a larger surface area and more variable morphology.
S. No |
Peak (cm-1) |
Functional Group |
---|---|---|
1 |
3444 |
-NH2 and -OH stretching primary amine |
2 |
2921 |
C–H stretch alkanes |
3 |
1631 |
C=C stretching alkene |
4 |
1094 |
C-O stretching aliphatic ether |
5 |
794 |
C=C bending alkene |
Zone of Inhibition (mm in diameter) |
|||
---|---|---|---|
Bacterial Strains |
Concentration |
||
0.01 g |
0.1 g |
Control |
|
Bacillus sp |
2.0 |
2.2 |
1.6 |
E.coli |
1.6 |
2.0 |
1.8 |
Enterobactersp |
2.1 |
2.6 |
1.8 |
Staphylococuus aureus |
2.6 |
2.9 |
1.8 |
Pseudomonas sp |
2.2 |
2.5 |
0.8 |
Absorbance spectra
UV-Visible spectroscopy is used to examine the optical properties of the CSNP-SiO2 nanocomposite, which are plotted in Figure 7. A UV-visible spectrum of CSNP-SiO2 nanocomposite revealed a broad absorption peak in the visible region at 380 nm. The adsorption behaviours at 380 nm indicate the presence of interenergetic bands between the conduction and valence bands of CSNPs, as well as the formation of CSNPs (Mragui, Zegaoui, & Silva, 2021). In other words, the wavelength difference of CSNP may be affected by its concentration (Saravanakumar & Dharmendirakumar, 2018). As a nucleation and stabiliser controller, the polysaccharide may control these changes in particle size and morphology.
Effect of Fluorescence Spectra
Figure 8 depicts the fluorescence spectra of a CSNP-SiO2 nanocomposite measured with an excitation wavelength. The Fluorescence (FL) band's centre appears at 608 nm. The fluorescence intensity increased as the size of the CSNP-SiO2 nanocomposite increased. The intensity of the fluorescence emission band and the absorption band of the CSNP-SiO2 nanocomposite were concentration and particle size dependent.
Thermogravimetric Analysis
The thermogravimetric analysis was used to assess the thermal stability of the composites. In a nitrogen atmosphere, the temperature of decomposition of the samples was investigated. TGA curves for chitosan and chitosan-SiO2 are shown in Figure 9. Thermal decomposition parameters were calculated using the TGA curves. Figure 9 shows data for CSNP-SiO2 nanocomposite from 50 to 650 °C at a heating rate of 10 °C/min. In general, all samples degrade in two stages: I water molecule loss and (ii) organic polymer material decomposition (Abbasi, 2017).
CSNP-SiO2 loses weight in two stages at temperatures ranging from 50 to 630 °C. The first weight loss (4.8mg) for CSNP-SiO2 occurred at 50–180 °C due to chitosan dehydration-induced evaporation of water molecules. Polymer chain decomposition is responsible for the second weight loss step (3.7 mg) at 200–420°C (Mahmoud, Nabil, Abdel-Aal, Fekry, & Osman, 2018). This shows that the chitosan/silica hybrid is completely involved in cross linking, and the results clearly show that it loses less weight than neat chitosan. The TGA plot clearly shows that chitosan and its composites degrade differently. The addition of the SiO2 inorganic particle to the biopolymer chitosan improved the thermal stability of the composites, according to TGA results.
Antibacterial Activity
Chitosan stabilizer, a naturally occurring polymer, was added in various amounts to the prepared silicon dioxide nanoparticles to produce Chitosan- Silicon dioxide with varying silicon dioxide percentages for long-term stability, prevention of nanoparticle agglomeration, and enhancement of antibacterial efficacy. The CSNP-SiO2 antibacterial activity was tested against five clinical pathogenic bacteria. Gram-positive Bacillus subtilis, Staphylococcus aureus, and Gram-negative Escherichia coli, Psedomonous, and Enterobacter strains were tested for antibacterial activity of the CSNP-SiO2 nanocomposite.Figure 10 depicts a distinct Zone of Inhibition (ZOI) surrounding the CSNP-SiO2 on culture-loaded MHA plates, indicating antibacterial efficacy. The mechanism underlying chitosan's antibacterial activity can be described as the interaction of positive charges in chitosan, such as protonated NH3 with negative charges in bacteria's cell walls via electrostatic forces. These interactions disrupt the function of the microbial cell membrane, preventing intracellular compounds from escaping and preventing nutrient transformation, ultimately killing the bacteria (Liu et al., 2006; Pasaribu, Kaban, Ginting, & Sinaga, 2018). Staphylococcus aureus and Enterobacter had the highest susceptibility at 24 and 48 hours, with zones of inhibition of 2.9 mm and 2.6 mm respectively. Psedomonous and Bacillus subtilis had the highest susceptibility to CSNP-SiO2 at 24 h, with zones of inhibition of 2.5 and 2.2 mm respectively, while Escherichia coli had the lowest zone of inhibition of 2.0 mm.
Conclusions
The properties of a novel chitosan-SiO2 nanocomposite were investigated after its successful synthesis. The XRD results show that increasing the spacing of SiO2 resulted in an intercalated structure. The FTIR spectra of all the composites show the presence of distinct organic and inorganic absorption bands. According to TGA results, the addition of chitosan-SiO2 nanocomposite particles to biopolymer chitosan improved the thermal stability of the composites. The prepared samples were tested for antibacterial activity against gram-positive Bacillus subtilis and Staphylococcus aureus bacteria strains as well as gram-negative Enterobacter, Escherichia coli, and Pseudomonas fluorescens bacteria strains using the suitable diffusion method. The results of the characterization demonstrated the formation of a CSNP-SiO2 nanocomposite. The antibacterial results confirmed that all the samples were antibacterial against the bacteria strains. Based on the findings, the CSNP-SiO2 nanocomposites could be an effective antibacterial agent against dangerous bacterial pathogens.