In silico predictions of NADPH oxidase binding properties of selected flavonoids - Protective role in arsenic induced neurotoxicity
Abstract
The study was an attempt to provide evidence for the effect of selected flavonoids on NADPH oxidase (NOX), which is a key enzyme in the generation of oxidative stress. For this study, a set of flavonoids were selected and screened for their molecular properties like physicochemical descriptions, oral bioavailability, Blood-Brain Barrier (BBB) penetration and P-glycoprotein (Pgp) substrate property using Molinspiration online tool, Swiss ADME and Swiss Target Prediction websites. Based on the molecular properties, three flavonoids (Naringenin, hesperidin and diosmin) were narrowed down for further molecular docking studies as they were found to be Pgp substrates. The protein targets were duly selected as they are the subunits of the NOX subtype, which was commonly expressed in the brain and they are key elements of NOX activation. Molecular docking studies were carried out with Autodock vina software to enumerate the binding energies of the flavonoid molecules with the selected protein targets. The three flavonoids naringenin, hesperidin and diosmin, have shown good binding energies for the selected targets compared to apocynin, a well-known NOX inhibitor. The molecular docking results revealed that hesperidin had shown the highest bonding energies, followed by diosmin and naringenin. These flavonoids naringenin, hesperidin and diosmin, might be the better inhibitors of NOX and might usefully help in combating arsenic induced neurotoxicity. These in silico findings need further confirmation by in vitro and in vivo studies.
Keywords
Arsenic neurotoxicity, NOX inhibition, flavonoids, in silico studies, molecular docking
Introduction
Arsenic is a heavy metal widely distributed in the environment as a pollutant through natural and anthropogenic sources. Naturally, arsenic leaches through the bedrocks of the earth's crust and anthropogenically through mining, agricultural pesticides and industrial wastage. Chronic arsenic exposure causes abnormalities from skin lesions to skin cancer. Additionally, it also causes cardiovascular, metabolic, neuronal and developmental disorders. The arsenic induced neurotoxicity is the point of concern in the current research and the mechanisms underlying arsenic induced neurotoxicity are not clear till date. Arsenic induced oxidative stress is the most accepted mechanism for arsenic toxicity; arsenic induced Reactive Oxygen Species (ROS) alters signalling pathways, mitochondrial function and enzyme activity 1. Arsenic was reported to activate several enzymes involved in ROS production like thioredoxin reductase, heme oxygenase reductase and NADPH oxidase (NOX) 2. Hence, the study was designed to screen the flavonoid compounds able to bind with NOX, the key mechanistic molecules responsible for arsenic induced ROS.
For many years, NOX was considered as a peculiarity of the scavenger cells. Several more homologues have been identified in the last decade and based on earlier studies, the NOX family is composed of the enzymes NOX1, NOX2, NOX3, NOX4, NOX5, DUOX1 and DUOX2 3. NOX is a membrane-bound ROS-producing enzyme involved in the electron transport chain and its overexpression is responsible for the initiation of oxidative stress. The subunits of NOX include P22phox, P40phox, gp91phox, P67phox, P47phox and Rac. A study demonstrated the activation of the P47phox subunit of NOX by arsenic in SVEC4‑10 endothelial cells 4 and also reported for phosphorylation and translocation of p67phox, p47phox, Rac1 in macrophages for generating superoxide radicals 5. In the case of arsenic-induced neurotoxicity excessive activation of NOX by arsenic plays an important role in the production of ROS and thus in oxidative stress production. Developing the new therapeutic interventions for arsenic induced neurotoxicity by suppressing NOX expression can be a substantial breakthrough in combating the toxic effects of arsenic.
Flavonoids, a group of natural substances with diverse phenolic structures, are found in fruits, vegetables, grains, bark, roots, stems, flowers, tea and wine. These natural products are known for their health benefits and flavonoids are now recognized as an essential ingredient in a variety of dietary supplements, pharmaceutical, medical and cosmetic applications. The antioxidant, anti-inflammatory, anti-mutagenic and anti-carcinogenic properties are coupled with their ability to regulate key cellular enzyme functions 6. In this study, few biologically active flavonoids were selected for predicting their NOX binding properties through in silico screening models.
In silico, drug discovery is emerging as a great tool which reduces research time and cost of drug discovery. In silico screening, studies were also useful for predicting the mechanism of action of various pharmacological compounds 7. This is one of the alternative techniques that can greatly reduce the usage of animals and have great sophistication with refined quality and efficacy. These days in silico tools are used as preliminary screening techniques for better designing of in vitro and in vivo experimentation. Hence, the current study was planned to evaluate selected flavonoids for their NOX binding properties using suitable in silico tools.
Methodology
Selection of flavonoids
A set of fourteen flavonoids, widely explored for their pharmacological properties, were selected from the pubmed (https://pubmed.ncbi.nlm.nih.gov/) to predict their NOX binding efficiency.
System requirements
Microsoft Windows 10 operating system with Intel Core i5 processor, 7th Gen with Dassault workstation and 16 GB of Ram system was used to carry out the in silico studies.
Prediction of molecular descriptors and bioactivity scores
Flavonoid International Union of Pure and Applied Chemistry (IUPAC) names and SMILES were obtained from PubChem (https://pubchem.ncbi.nlm.nih.gov/). The molecular descriptors log P, topological surface area, molecular weight, number of hydrogen bond donors and acceptors and number of violations were calculated. Then bioactivity scores for G-protein coupled receptor (GPCR) ligand, ion channel modulator, kinase inhibitor, protease inhibitor, nuclear receptor ligand and enzyme binding properties were predicted with Molinspiration online tool (http://www. molinspiration. com/cgi-bin/properties).
Prediction of BBB permeability, Pgp substrate affinity and targets
Blood Brain Barrier (BBB) permeation and P-glycoprotein (Pgp) substrate property were computed using the Swiss ADME web service (http://www. swissadme. ch/). The target prediction studies were performed to determine the suitable target for the selected flavonoids using swiss target prediction (http://www.swiss target prediction), a web server which accurately predicts the targets of bioactive molecules using a combination of 2D and 3Dsimilarity measures with known ligands.
Target selection, ligand preparation and docking
Crystallographic structures of the NOX subunits were obtained from the protein data bank (https://www.rcsb.org/). The protein targets were i) Crystal structure of NADPH binding domain of gp91 (phox) ii) Structure of the p22phox-p47phox complex iii) Rac1-RhoGDI complex involved in NADPH oxidase activation iv) Hetero dimer of p40phox and p67phox PB1 domains from human NADPH oxidase and v) SH3 domain of NADPH oxidase activator 1 [PDB ID: 3A1F, 1OV3, 1HH4, 1OEY and 7CFZ] (Table 2).
S.No. |
Flavonoid |
IUPAC name |
SMILES |
PubChem ID |
---|---|---|---|---|
1. |
Naringenin |
5,7-dihydroxy-2-(4-hydroxyphenyl)-2,3-dihydrochromen-4-one |
C1C(OC2=CC(=CC(=C2C1=O)O)O) C3=CC=C(C=C3)O |
932 |
2. |
Hesperidin |
(2S)-5-hydroxy-2-(3-hydroxy-4-methoxyphenyl)-7-[(2S,3R,4S,5S,6R)-3,4,5-trihydroxy-6-[(2R,3R,4R,5R,6S)-3,4,5-trihydroxy-6-methyloxan-2-yl]oxymethyl]oxan-2-yl]oxy-2,3-dihydrochromen-4-one |
C[C@H]1[C@@H]([C@H] ([C@H]([C@@H](O1)OC[C@@H] 2[C@H]([C@@H]([C@H]([C@@H (O2)OC3=CC(=C4C(=O)C[C@H](OC4=C3) C5=CC(=C(C=C5)OC)O)O)O)O)O)O)O)O |
10621 |
3. |
Diosmin |
5-hydroxy-2-(3-hydroxy-4-methoxyphenyl)-7-[(2S,3R,4S,5S,6R)-3,4,5-trihydroxy-6-[[(2R,3R,4R,5R,6S)-3,4,5-trihydroxy-6-methyloxan-2-yl]oxymethyl]oxan-2-yl]oxychromen-4-one |
C[C@H]1[C@@H]([C@H]([C@H] ([C@@H(O1)OC[C@@H]2[C@H] ([C@@H]([C@H]([C@@H](O2)OC3=CC (=C4C(=C3)OC(=CC4=O)C5=CC (=C(C=C5)OC) O)O)O)O)O)O)O)O |
5281613 |
4. |
Luteolin |
2-(3,4-dihydroxyphenyl)-5,7-dihydroxychromen-4-one |
C1=CC(=C(C=C1C2=CC(=O)C3=C(C=C (C=C3O2)O)O)O)O |
5280445 |
5. |
Resveratrol |
5-[(E)-2-(4-Hydroxyphenyl) ethen-1-yl]benzene-1,3-diol |
C1=CC(=CC=C1C=CC2=CC(=CC(=C2) O)O)O |
445154 |
6. |
Quercetin |
2-(3,4-dihydroxyphenyl)-3,5,7-trihydroxychromen-4-one |
C1=CC(=C(C=C1C2=C(C(=O)C3=C (C=C(C=C3O2)O)O)O)O)O |
5280343 |
7. |
Kaempferol |
3,5,7-trihydroxy-2-(4-hydroxyphenyl) chromen-4-one |
C1=CC(=CC=C1C2=C(C(=O)C3=C (C=C(C=C3O2)O)O)O)O |
5280863 |
8. |
Taxifolin |
(2R,3R)-2-(3,4-dihydroxyphenyl)-3,5,7-trihydroxy-2,3-dihydrochromen-4-one |
C1=CC(=C(C=C1[C@@H]2[C@H](C(=O) C3=C(C=C(C=C3O2)O)O)O)O)O |
439533 |
9. |
Eriodictyol |
(2S)-2-(3,4-dihydroxyphenyl)-5,7-dihydroxy-2,3-dihydrochromen-4-one |
C1[C@H](OC2=CC(=CC(=C2C1=O)O)O) C3=CC(=C(C=C3)O)O |
440735 |
10. |
Fisetin |
2-(3,4-dihydroxyphenyl)-3,7-dihydroxychromen-4-one |
C1=CC(=C(C=C1C2=C(C(=O)C3=C(O2) C=C(C=C3)O)O)O)O |
5281614 |
11. |
Galangin |
3,5,7-trihydroxy-2-phenylchromen-4-one |
C1=CC=C(C=C1)C2=C(C(=O)C3=C (C=C(C=C3O2)O)O)O |
5281616 |
12. |
Genistein |
5,7-dihydroxy-3-(4-hydroxyphenyl) chromen-4-one |
C1=CC(=CC=C1C2=COC3=CC (=CC(=C3C2=O)O)O)O |
5280961 |
13. |
Isorhamnetin |
3,5,7-trihydroxy-2-(4-hydroxy-3-methoxyphenyl) chromen-4-one |
COC1=C(C=CC(=C1)C2=C(C(=O)C3=C (C=C(C=C3O2)O)O)O)O |
5281654 |
14. |
Myricetin |
3,5,7-trihydroxy-2-(3,4,5-trihydroxyphenyl) chromen-4-one |
C1=C(C=C(C(=C1O)O)O)C2=C(C(=O) C3=C(C=C(C=C3O2)O)O)O |
5281672 |
S.No. |
Target protein |
PDB ID |
---|---|---|
1. |
The crystal structure of NADPH binding domain of gp91(phox) |
3A1F |
2. |
Structure of the p22phox-p47phox complex |
1OV3 |
3. |
Rac1-RhoGDI complex involved in NADPH oxidase activation |
1HH4 |
4. |
Hetero dimer of p40phox and p67phox PB1 domains from human NADPH oxidase |
1OEY |
5. |
SH3 domain of NADPH oxidase activator 1 |
7CFZ |
S.No. |
Descriptors |
Milog P |
TPSA |
m.wt |
nON |
nOHNH |
n rot |
---|---|---|---|---|---|---|---|
1. |
Naringenin |
2.12 |
86.99 |
272.26 |
3 |
5 |
1 |
2. |
Hesperidin |
-0.55 |
234.30 |
610.57 |
15 |
8 |
7 |
3. |
Luteolin |
1.97 |
111.12 |
286.24 |
6 |
4 |
1 |
4. |
Resveratrol |
2.99 |
60.68 |
228.25 |
3 |
3 |
2 |
5. |
Quercetin |
1.68 |
131.35 |
302.24 |
7 |
5 |
1 |
6. |
Kaempferol |
2.17 |
111.12 |
286.24 |
6 |
4 |
1 |
7. |
Diosmin |
2.53 |
109.36 |
330.29 |
7 |
3 |
3 |
8. |
Taxifolin |
0.71 |
127.44 |
304.25 |
7 |
5 |
1 |
9. |
Eriodictyol |
1.20 |
107.22 |
288.25 |
6 |
4 |
1 |
10. |
Fisetin |
1.97 |
111.12 |
286.24 |
6 |
4 |
1 |
11. |
Galangin |
2.65 |
90.89 |
270.24 |
5 |
3 |
1 |
12. |
Genistein |
2.27 |
90.89 |
270.24 |
5 |
3 |
1 |
13. |
Isorhamnetin |
1.99 |
120.36 |
316.26 |
7 |
4 |
2 |
14. |
Myricetin |
1.39 |
151.58 |
318.24 |
8 |
6 |
1 |
MiLog P- Logarithm of partition coefficient between n-octanol and water; TPSA- Topological polar surface area; nON- Number of hydrogen bond acceptors; nOHNH-Number of hydrogen bond donors; nrot- Number of rotatable bonds; m.wt- Molecular weight
S.No. |
Descriptors |
GPCR |
Ion channel modulator |
Kinases |
Nuclear receptor |
Protease inhibitor |
Enzyme binding |
---|---|---|---|---|---|---|---|
1. |
Naringenin |
0.03 |
-0.20 |
-0.26 |
0.42 |
-0.12 |
0.21 |
2. |
Hesperidin |
-0.01 |
-0.59 |
-0.36 |
-0.20 |
0.00 |
0.06 |
3. |
Luteolin |
-0.02 |
-0.07 |
0.26 |
0.39 |
-0.22 |
0.28 |
4. |
Resveratrol |
-0.20 |
0.02 |
-0.20 |
0.01 |
-0.41 |
0.02 |
5. |
Quercetin |
-0.06 |
-0.19 |
0.28 |
0.36 |
-0.25 |
0.28 |
6. |
Kaempferol |
-0.10 |
-0.21 |
0.21 |
0.32 |
-0.27 |
0.26 |
7. |
Diosmin |
-0.12 |
-0.28 |
0.21 |
0.23 |
-0.27 |
0.18 |
8. |
Taxifolin |
0.09 |
0.03 |
-0.04 |
0.29 |
0.05 |
0.29 |
9. |
Eriodictyol |
0.05 |
0.03 |
-0.07 |
0.26 |
0.03 |
0.29 |
10. |
Fisetin |
-0.11 |
-0.27 |
0.18 |
0.20 |
-0.36 |
0.20 |
11. |
Galangin |
-0.13 |
-0.21 |
0.19 |
0.28 |
-0.32 |
0.28 |
12. |
Genistein |
-0.22 |
-0.54 |
-0.06 |
0.23 |
-0.68 |
0.13 |
13. |
Isorhamnetin |
-0.10 |
-0.26 |
0.25 |
0.28 |
-0.30 |
0.22 |
14. |
Myricetin |
-0.06 |
-0.18 |
0.28 |
0.32 |
-0.20 |
0.30 |
S.No. |
Flavonoids |
BBB permeability |
PGP substrate |
---|---|---|---|
1. |
Naringenin |
No |
Yes |
2. |
Hesperidin |
No |
Yes |
3. |
Luteolin |
No |
No |
4. |
Resveratrol |
Yes |
No |
5. |
Quercetin |
No |
No |
6. |
Kaempferol |
No |
No |
7. |
Diosmin |
No |
Yes |
8. |
Taxifolin |
No |
No |
9. |
Eriodictyol |
No |
No |
10. |
Fisetin |
No |
No |
11. |
Galangin |
No |
No |
12. |
Genistein |
No |
No |
13. |
Isorhamnetin |
No |
No |
14. |
Myricetin |
No |
No |
S.No. |
Ligand |
Docking Scores (Kcal/mol) |
Interacting amino acids |
Nature of bonds |
---|---|---|---|---|
1. |
Naringenin |
-6.1 |
Asn49, Asn46, Lys42, Phe7 |
Vanderwaals-6, CHB-1 |
2. |
Hesperidin |
-7.4 |
Asn93, Gln123, Tyr56, Unk0, Lys54, Lys53, Ile139, His143. |
CHB-1 |
3. |
Diosmin |
-7.3 |
Ala87, Leu90, gln142, Glu135, Tyr58, Asn193 |
CHB-5 |
4. |
Apocynin |
-4.6 |
Asn 169, Val 177, Ala 140 |
CHB-1 |
S.No. |
Ligand |
Docking score (Kcal/mol) |
Interacting amino acids |
Nature of bonds |
---|---|---|---|---|
1. |
Naringenin |
-6.8 |
Leu158, Gln197, Val186, Ile156, Ser152, Pro153. |
CHB-1 |
2. |
Hesperidin |
-8.7 |
Ser152, Phe155, Ile156, Pro153, Ile156, Gln197, Leu158, Met198, Glu184 |
CHB-4 |
3. |
Diosmin |
-8.5 |
Ser152, Pro153, Phe155, Ile156, Leu158, Glu184, Gln197 |
CHB-4 |
4. |
Apocynin |
-5.5 |
Trp 193, Tyr 167,Cys 196, Met 175, Leu 177 |
CHB-1 |
S.No. |
Ligand |
Docking score (Kcal/mol) |
Interacting amino acids |
Nature of bonds |
---|---|---|---|---|
1. |
Naringenin |
-6.8 |
Leu158, Gln197, val186, Ile156, Pro153, Ser152. |
CHB-1 |
2. |
Hesperidin |
-8.7 |
Ser152, Phe155, Ile156, Pro153, Gln197, Leu158, Met198, Glu184. |
CHB-4 |
3. |
Diosmin |
-8.5 |
Ser152, Phe155, Ile156, Pro153, Gln197, Leu158, Met198, Glu184. |
- |
4. |
Apocynin |
-4.3 |
Arg102, Gly 150 |
CHB-1 |
S.No. |
Ligand |
Docking scores (Kcal/mol) |
Interacting amino acids |
Nature of bonds |
---|---|---|---|---|
1. |
Naringenin |
-7.4 |
Leu313, Phe320, Leu319,, Lys323, Glu258, Leu273, Val257 |
CHB-2 |
2. |
Hesperidin |
-9.2 |
Glu277, Asp330, Gln328, Lyr244, Arg280, Asp247. |
CHB-3 |
3. |
Diosmin |
-9.3 |
Gln279, Glu277, Phe278, Asn331, Glu246, Asp247, Tyr244. |
CHB-2 |
4. |
Apocynin |
0 |
0 |
0 |
S.No. |
Ligand |
Docking scores (Kcal/mol) |
Interacting amino acids |
Nature of bonds |
---|---|---|---|---|
1. |
Naringenin |
-6.2 |
Ca501, Asp130, Asp134 |
- |
2. |
Hesperidin |
-7.4 |
Asp130, Ca501, Asp134. |
- |
3. |
Diosmin |
-6.6 |
Asp130, Ca501, Asp134. |
Vanderwalls-6 |
4. |
Apocynin |
0 |
0 |
0 |
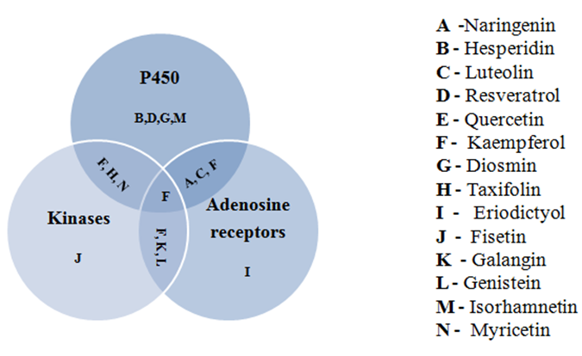
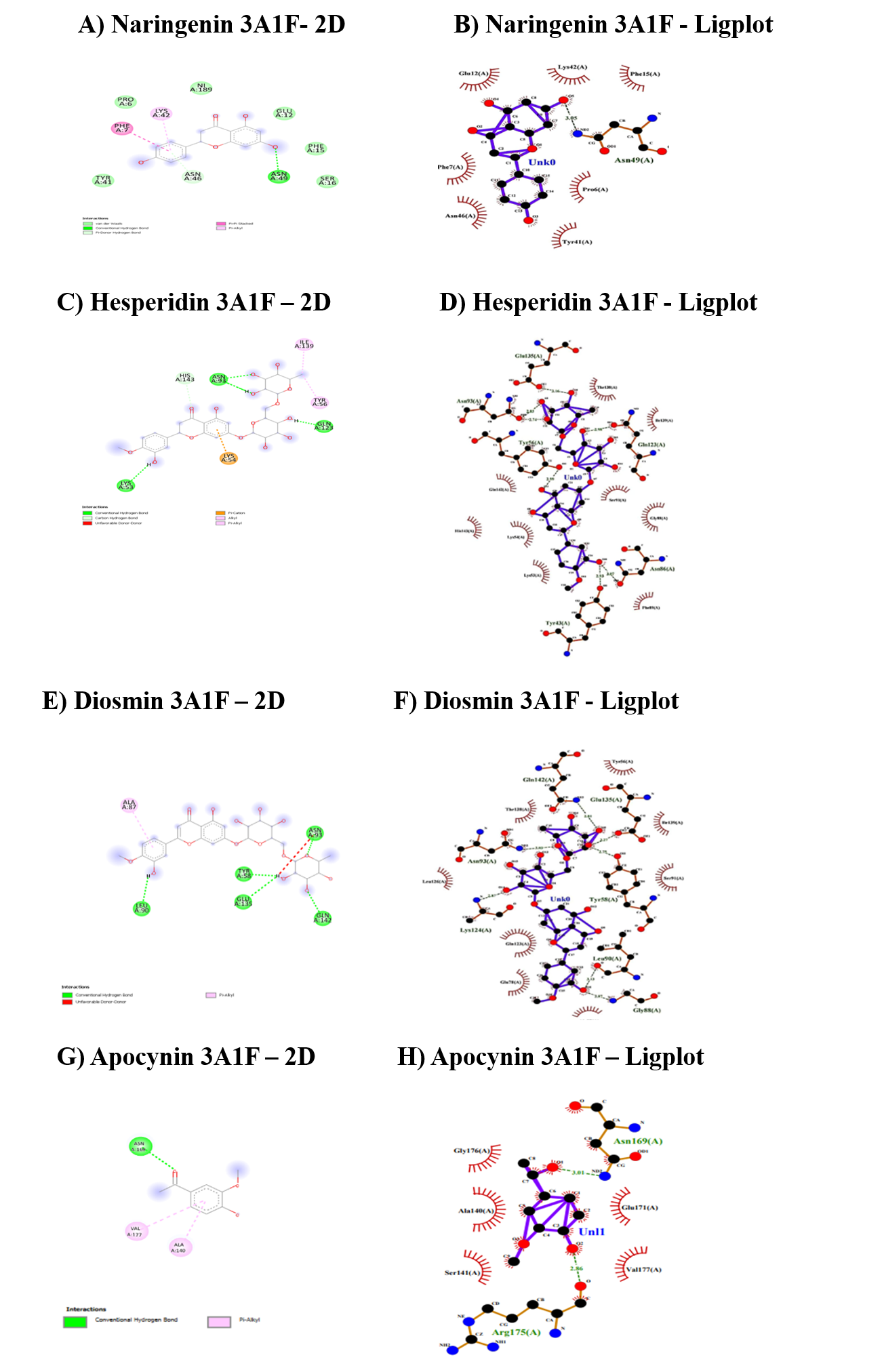
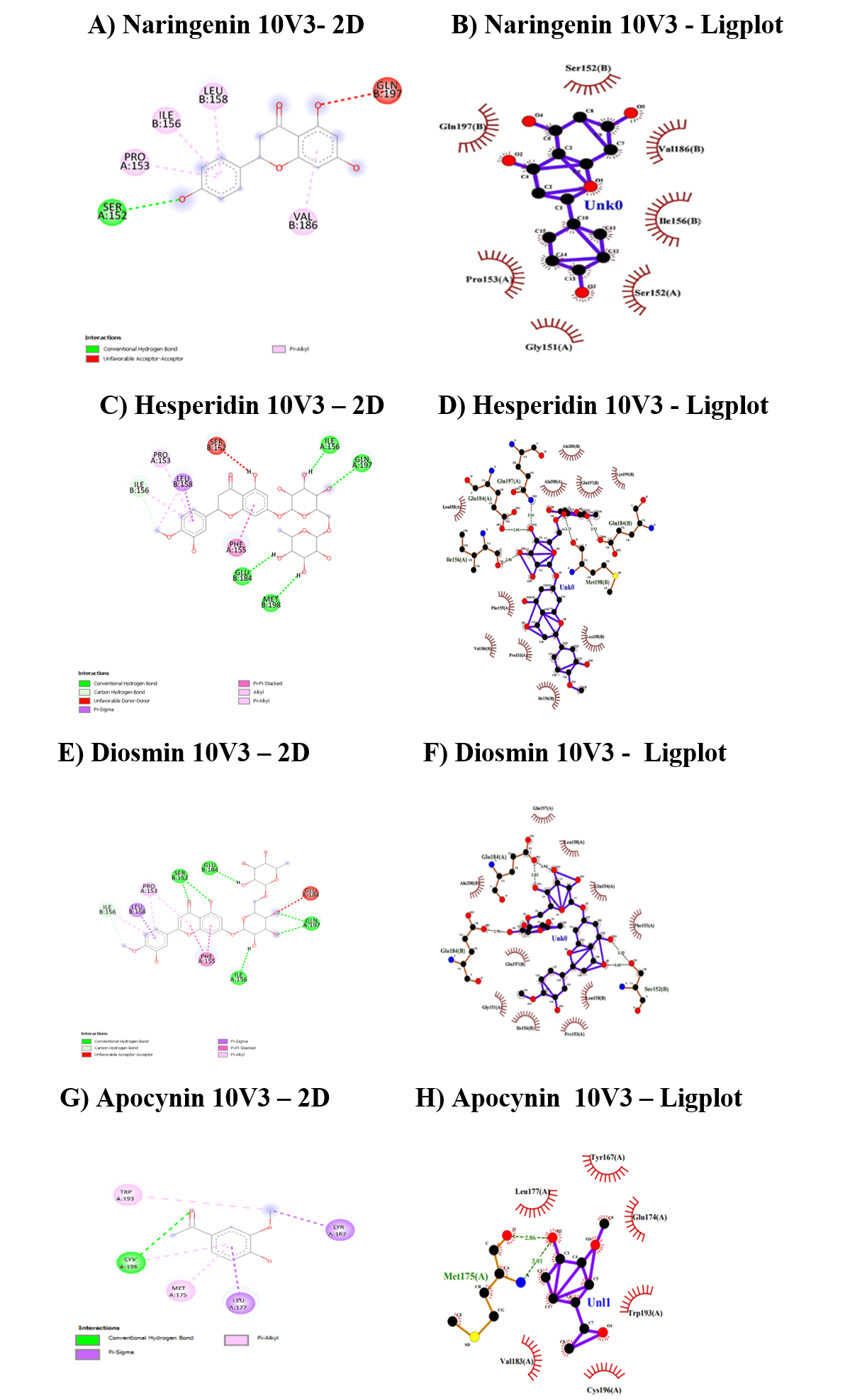
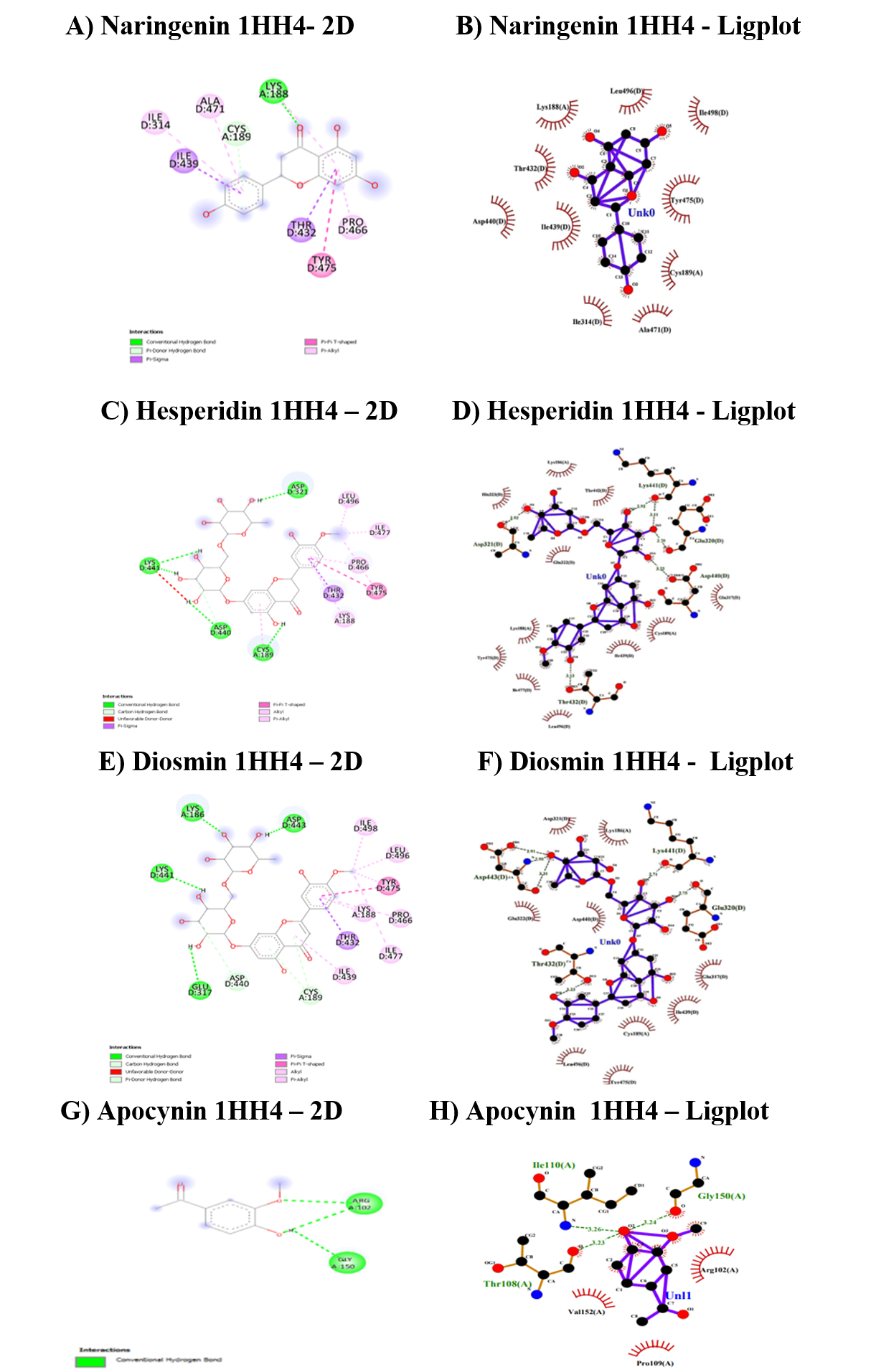
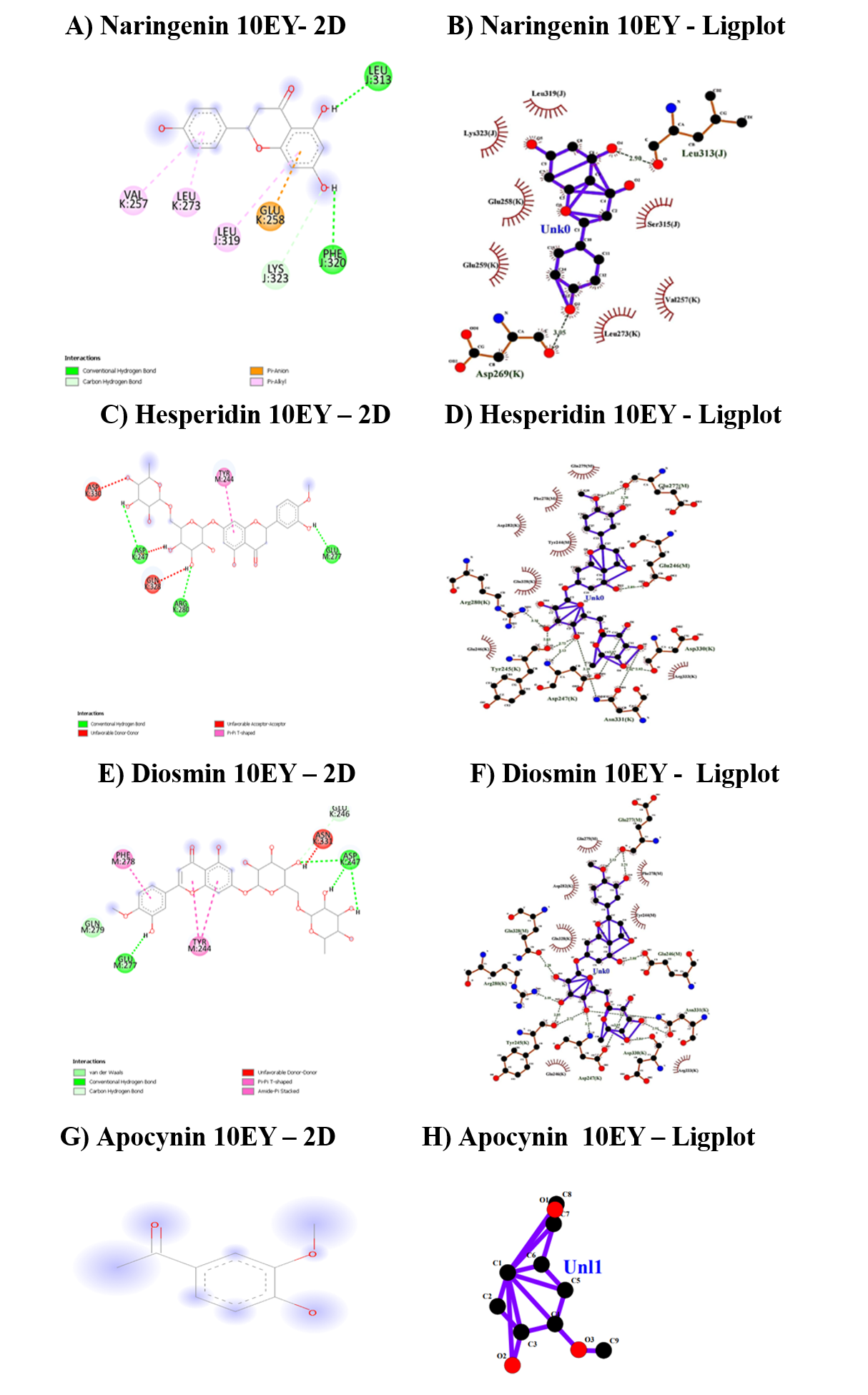
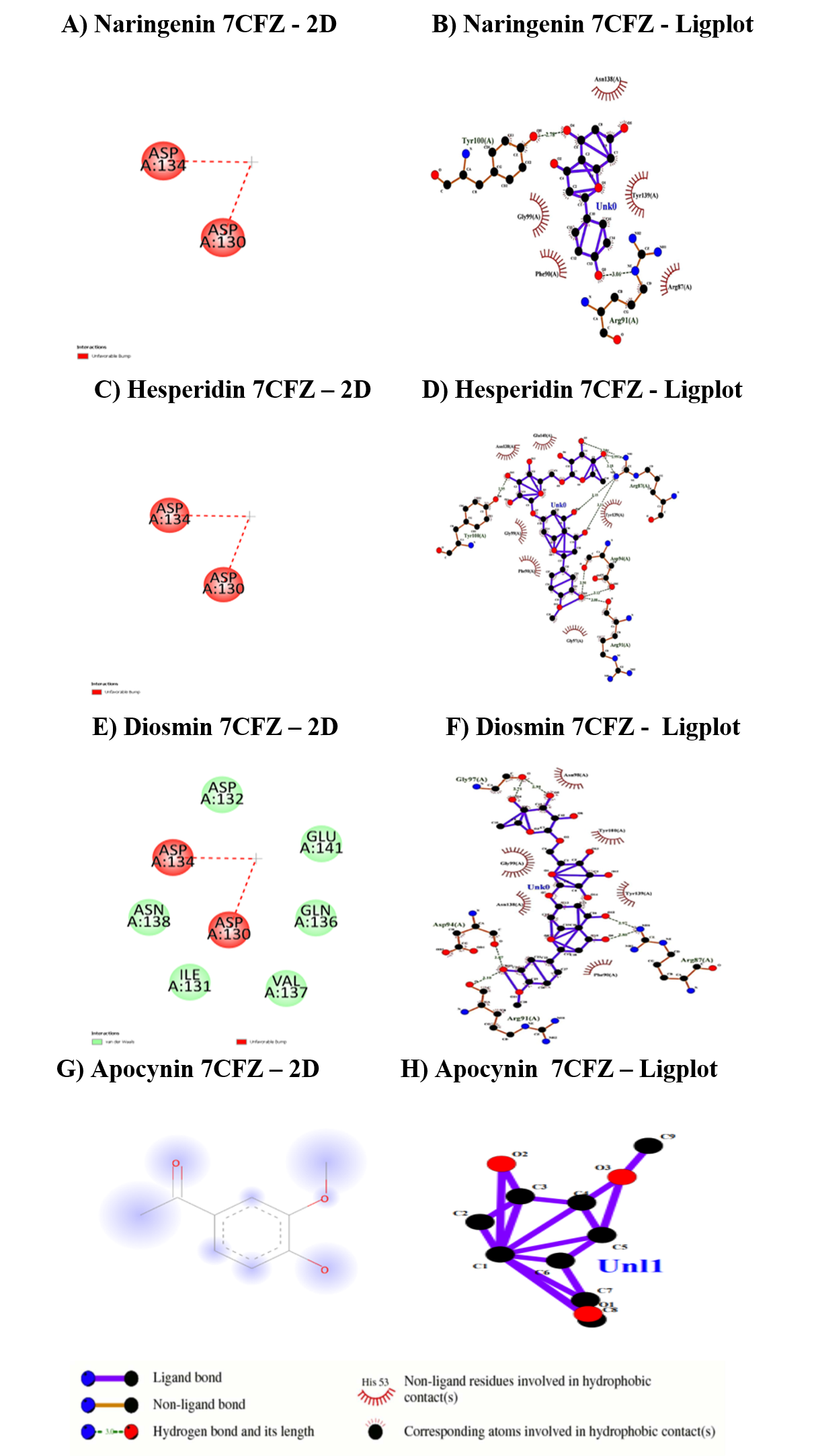
The binding site includes all residues within the 2.0°A vicinity of the ligand. Three flavonoids were selected from the fourteen flavonoids based on the physicochemical and biological properties and processed for molecular docking studies. Chemical structures were drawn with the application Chem and Bio Draw 12.0 and saved as mol2 files using Chem and Bio 3D version 12.0. Table 1 represents the structures and IUPAC nomenclature of the flavonoids. Autodock Vina (https://autodock.scripps.edu/downloads)was used for docking and MGL Tools (https://autodock.scripps.edu/downloads) were used for visualization and analysis of molecular structures. Discovery Studio Visualizer (https://discover.3rds.com/discovery-studio) was used for protein and modeling data analysis. Then Pymol (https://pymol.org/2/) exhibited high-quality molecular graphics and animations. Ligplot (https://www.ebi.ac.uk/thornton-srv/software/LIGPLOT) generated schematic 2D representations of protein-ligand complexes. The binding energies, interacting amino acids, and nature of bonding were predicted and the best docking scores were noted down.
Results and Discussion
In silico molecular descriptor studies
The selected flavonoids were tested for Lipinski's rule of five, which generally tests drug-likeness, i.e. orally active in humans. As per Lipinski's rule, the Partition coefficient log P ranges from −0.4 to+5.6, and molar refractivity ranges from 40 to 130, molecular weight ranges from 180 to 500, number of atoms from 20 to 70 (including H-bond donors and H-bond acceptors). The polar surface area must be not greater than 140°A to have good oral bioavailability. The compounds Log P scores were in the following range of -0.55 to 2.99, a molecular weight of 228.25–330.29 Daltons, Hydrogen Bond Acceptor (HBA) values of 3–8 and Hydrogen Bond Donor (HBD) values of 1–7. All the compounds followed Lipinski rule, but the molecular weight of hesperidin (610.57 Daltons) was slightly higher than the Lipinski range and remaining all the selected flavonoids have good oral bioavailability (Table 3).
The bioactivity scores were predicted, and if the compound scores 0.00 indicates its high activity, if it scores −0.50 to 0.00 represent moderate activity and if it is ≥0.50 indicates poor activity. The bioactivity scores for the GPCR ligand binding of the selected flavonoid compounds were found, ranging from -0.01 to 0.09, indicating moderate to high GPCR ligand binding capacity. The binding scores for other receptor molecules like ion channels, kinases, nuclear receptors, and protease enzymes were found to be ranging from -0.59 to 0.39, indicating poor activity on those receptors (Table 4 and Table 5 ).
When tested for BBB permeability and Pgp substrate property, resveratrol had good BBB permeability, whereas naringenin, hesperidin and diosmin were found to be Pgp substrates. In the target prediction studies, flavonoids naringenin, luteolin, hesperidin, taxifolin, resveratrol, diosmin, quercetin, kaempferol, and isorhamnetin were most likely to bind with P 450 enzyme. Taxifolin, quercetin, myrcetin, kaempferol, genistein, galangin and fisetin were intended to bind with kinases. Naringenin, luteolin, kaempferol, genestein, galangin and Eriodictyol were predicted to bind with adenosine receptors (Figure 1).
Due to their good physicochemical and biological properties, three flavonoids, diosmin, hesperidin and naringenin, were selected for the further molecular docking studies. Diosmin, hesperidin and naringenin were found to be the substrates of Pgp, which can enhance the BBB crossing permeability, as Pgp is a key element of the BBB which actively transport various lipophilic drugs. These flavonoids also exhibited good P450 enzyme binding properties in target prediction studies. Diosmin, naringenin and hesperidin were then docked with the subunits of the NOX subtype which were predominantly expressed in the brain. Apocynin, an established NOX inhibitor, was taken as a standard to compare the test molecules.
The interaction summary of the NADPH binding domain of gp91phox (3A1F) was given in Table 6 and Figure 2. From the results, it was clear that hesperidin has been found to have higher binding efficiency followed by diosmin and naringenin and hence hesperidin can better interact with the NADPH binding domain of gp91phox. Hesperidin and diosmin formed conventional hydrogen bonds (CHB) bonds with the target, whereas naringenin formed both CHB and vander waals. When compared to apocynin, all the three flavonoids have exhibited a better docking score and the nature of bonding was also similar to that of standard, i.e., CHB bonds. The binding regions to the target for all the flavonoids ranged from Phe7 to Asn193.
The second target studied was the P22Phox-P47Phox complex (10V3) and the interaction summary was illustrated in Table 7 and Figure 3. Again, for this target, hesperidin has recorded with the highest docking score of -8.7 kcal/mol with CHB and covalent hydrogen bonds, followed by diosmin and naringenin.
The docking scores for all three flavonoids were higher than the apocynin. Diosmin, naringenin and apocynin interacted with the amino acids in the docking using CHD. The binding regions of all flavonoids ranged from Ser152 to Met198.
The interaction energies, amino acids and nature of bonds for the Rac1-RhoGD complex involved in NADPH oxidase activation (1HH4) with flavonoids were given in Table 8 and Figure 4. The binding affinity for hesperidin was found to be higher than diosmin and naringenin. The three flavonoids exhibit higher bonding energies than the standard apocynin and the binding regions, ranging from Arg 102 to Met198. The nature of the bonds was CHB for naringenin and hesperidin, similar to apocynin, but diosmin does not exhibit CHB.
The fourth target protein was the Hetero dimer of P40Phox and P67Phox PB1domains from human NADPH oxidase (10EY) and the details bonding with flavonoids are given in Table 9 and Figure 5. When the docking scores were compared, diosmin exhibited the highest score than hesperidin and naringenin. All three flavonoids interacted with the CHB bonding, whereas apocynin has not shown any interaction with this protein target and the binding region lies between Tyr244 to Asn331.
The binding affinities for the target SH3 domain of NADPH oxidase activator 1 (7CFZ) were illustrated in Table 10 and Figure 6. Hesperidin was shown higher bonding affinity than diosmin and naringenin where, as apocynin has shown zero affinity for the protein target. The binding range lies between Asp130 to Ca501. The diosmin exhibit only vanderwaal interactions no CHB existed.
Apocynin, in a study, binding with NADPH binding domain of gp91phox (3A1F) was predicted to prevent ischemic stroke 8. Hesperidin inhibition of p22phox and p47phox subunits of NOX in the aorta was responsible for its anti-hypertensive effect 9. P22phox subunit of NOX4 inhibited by naringenin has shown the suppression of oxidative stress and inflammatory response 10. Curcumin was reported to alleviate the expression of Rac1, gp91 and p47phox, causes suppression of diabetic cardiomyopathy 11. Naringenin ameliorated nephrotoxicity induced by daunorubicin via modulating the expression of p67 phox 12. Quercetin suppressed p67phox in a study on high-fat diet-induced atherosclerosis indicating the role of p67phox in inducing atherosclerosis 13. The above cited studies revealed the other possible activities of the selected targets apart from the neuroprotective effect.
Conclusion
The above docking results revealed that hesperidin had recorded higher binding energies compared to two other flavonoids, followed by diosmin and naringenin. Hesperidin has shown its strongest binding to Hetero dimer of P40Phoxand P67Phox PB1domains from human NADPH oxidase (10EY). As the selected protein targets were specially expressed in the brain, the flavonoids naringenin, hesperidin and diosmin might be good drug candidates to treat neurotoxicity, neurodegeneration etc. Further mechanisms can be revealed by carrying out in vitro and in vivo studies to determine the NOX inhibiting properties in the living systems.
Conflict of Interest
The authors declare that they have no conflict of interest.
Funding Support
The authors are thankful to UGC-NFSC scheme for providing funding for Rupasree Peruru to carry out the study.