Characterization of Methotrexate Loaded Fucoidan/Chitosan Nanoparticles
Abstract
Methotrexate is one of the most popular and safe anti-inflammatory drugs which is an antifolate-type antimetabolite and is used as an anticancer drug. In this study, oppositely charged chitosan and fucoidan have been non-covalently bonded using their electrostatic interactions with the methotrexate drug loaded into these nanoparticles. Fucoidan is obtained from marine algae which are composed of l-fucose and sulfate groups in various types of brown seaweeds; whereas chitosan is a naturally occurring biopolymer obtained through the N-deacetylation of chitin. Depending on the fucoidan / chitosan (F / C) weight ratio, three distinct nanoparticles (1F / 1C; 3F / 1C; 5F / 1C) are synthesized and characterized. Nanoparticles were prepared using cross linkers EDC and NHS at a constant pH to reduce the conjugate size. The prepared conjugates were characterized for their size and zeta potential using DLS analysis and the functional groups were analysed using FTIR. DLS results proclaimed that there was size reduction in particle size with cross linker and without the drug methotrexate. The 5:1 F/C nanoparticles was seen to be 441.5 nm, the difference being considerable larger in the 5:1 formulation. Hence for further analysis 5:1 F/C nanoparticles were preferred. Maximum entrapment efficiency was observed in 5:1 F/C nanoparticle with and without cross linkers. To understand the structural morphology of nanoparticles electromagnetic magnification like SEM and TEM were taken in account.
Keywords
Fucoidan, sulfated polysaccharides, chitosan, methotrexate, anti-cancer
Introduction
Since ancient time’s, humans have widely considered using plant-based natural products as medicines against various diseases. Modern medicines are extracted from herbs using conventional knowledge and practices. These natural product-based drug discoveries have numerous advantages but are not preferred for drug discovery and drug delivery systems when compared to commercially available chemical compounds. Despite all this, natural compounds are currently being considered and studied for the treatment of a number of major diseases, including diabetes, cancer, inflammatory responses and cardiovascular disease (Patra et al., 2018).
In the circulatory system, nanostructures last longer and allow the release of synthetic drugs as per the prescribed dosage. The intake of these particles by cells is much higher than that of larger particles. Therefore, a decrease in plasma fluctuations and reduced side effects are observed. Drug nanoparticles show increased bioavailability, increased ability to cross the blood-brain barrier (BBB), and enter the pulmonary system to absorb by a lining of endothelial cells. Therefore, they work directly together to treat diseased cells or affected cells with greater efficacy with minimum adverse effects.
Nanotechnology has been shown to remove the barrier between biological and physical sciences through the application of nanostructures and nanophases. The Nanobiotechnological field increased the exploitation of marine resources and also important in a variety of areas, such as biomedicine, cosmetics, and food. Marine polysaccharide-based materials have recently gained attention due to their biocompatibility, biodegradability, low cost and abundance availability (Coutinho, Barbosa, & Reis, 2019). These substances can be found in a number of biological sources, such as algae, animals and micro-organisms; they include a wide complex group of different macromolecules which have a variety of biological applications and interesting features that can be used to build a drug delivery system. This approach to nanoparticle design and delivery of drugs in a highly targeted, efficient manner with less adverse effects on patients can be considered for drug delivery systems (Barbosa, Lima, & Reis, 2019; Patra et al., 2018).
Fucoidan is a fucose-rich sulphated polysaccharide obtained mainly from brown seaweed. It is composed of l-fucose, sulphate groups and small proportions of d-xylose, d-mannose, d-galactose, l-rhamnose, arabinose, glucose, d-glucuronic acid and acetyl groups. It has many different biological properties such as anti-coagulant, anti-thrombotic, anti-angiogenic, anti-proliferative and anti-cancer. The enzyme Fucoidanase is responsible for fucoidan hydrolysis and follows key pathways such as PI3K / AKT, MAPK pathway, and caspase pathway (Ana Isabel Barbosa et al., 2019; Aquib et al., 2019; Wu et al., 2016).
It uses chemical reactions, inactive groups, such as carboxylic, sulphate and hydroxyl groups, which help to improve the performance of different drugs, in particular, their absorption and solvent properties. Polysaccharides such as fucoidan, carrageenan, alginate and chitosan have special medicinal properties, such as biocompatibility, biodegradability and bioactivity, and have been extensively investigated for their efficacy against breast and lung cancer (Aquib et al., 2019). Fucoidan combines its function through a variety of mechanisms such as cell cycle arrest, apoptosis and immune function; and anti-cancer properties that include inflammation, oxidative stress, immune system and stem cell stimulation (Wu et al., 2016).
Chitosan is an interesting polymer commonly used in the field of medicine and science. It is a naturally occurring biopolymer formed by the N-deacetylation of chitin. Chitin is found in the exoskeleton of marine crustaceans, including shrimp and crab. This polysaccharide has the characteristic of mucoadhesion and facilitates the transport of paracellular drugs via the transient opening of tight junctions between epithelial cells. As chitin occurs naturally, chitosan is a completely biodegradable and biocompatible compound and can therefore be used as an adhesive as well as an antibacterial and antifungal agent (Lin et al., 2007). These properties of chitosan are valuable to the drug delivery system. Oppositely charged chitosan and fucoidan have been studied and their electrostatic interactions have led to an increase in the use of polyelectrolyte complexation in nanoparticle-based delivery systems for the delivery of growth factor drugs and other biological compounds (Coutinho et al., 2019; Ekinci, Ilem-Ozdemir, Gundogdu, & Asikoglu, 2015).
Methotrexate (MTX) is a well-known drug used to treat and maintain control over cancers like leukaemia, osteosarcoma etc. This compound impedes dihydrofolate reductase (DHFR), an enzyme that takes part in the tetrahydrofolate synthesis. However, there are two major barriers in MTX bioavailability and clinical efficacy: its poor solubility in an aqueous solution; and its rapid degradation in the intestinal tract. The lymphatic system plays a salient feature in cancer metastasis and immune response. MTX is an anti-cancer and immune-related agent and is delivered to the immune system through the lymphatic system. The lymphatic system uses the transfer of nano drugs through diffusion into the intercellular space of lymphatic walls. MTX integration into nanoparticles will solve drug shortages as well as enhance its stability and bioactivity issues (Barbosa et al., 2019; Ekinci et al., 2015; Vakilinezhad, Amini, Dara, & Alipour, 2019).
Cross-linking agents like (EDC/NHS) are used to improve stability and minimise the size of nanoparticles. EDC is also used to cross-link amines (NH2) to carboxylate groups; this activity being improved by the NHS through the activation of carboxylate groups. EDC is the most widely recognised zero-length cross-linker for biochemical conjugation as it can efficiently form conjugates between two molecules, protein and oligonucleotide, protein and peptide, and small molecules. As EDC is used on its own, the reaction between the EDC and the hydroxyl groups produces an unstable o-acylisoure-based intermediate that is undergoing hydrolysis in the presence of water. The NHS is useful for stabilising the intermediate functional group before it reacts with its counterpart. This combination of crosslinkers is also preferred for the processing of some nanoparticles.
Targeted nanocarriers have been used in recent years to improve the bio-distribution, pharmacological, therapeutic and toxic properties of agents used in cancer diagnosis and therapy. As a result, nanocarriers have been commonly used for the early detection and treatment of cancer (Ekinci et al., 2015). The aims of this study are to synthesise and characterise methotrexate-charged fucoidan-chitosan nanoparticles.
Materials and Methods
Reagents and chemicals
Fucoidan (MW 50.00 to 190.00 Da) from Fucus vesiculosus- Sigma Aldrich (Brahma Science, India), Chitosan (MW 190.00 to 310.00 Da) from Sigma Aldrich (Brahma Science, India), Acetic acid solution 1% (v/v), Double deionised water, MTX 15% (w/w) from Sigma Aldrich (Brahma Science, India)), 1-Ethyl-3-(3-dimethylaminopropyl) carbodiimide (EDC) and N-hydrosucinamide (NHS) from SRL India.
Preparation of fucoidan/chitosan nanoparticles
The polyelectrolyte self-assembly method was used to prepare the Fucoidan/chitosan (F/C) nanoparticles using the ultrasonication technique at room temperature. Basically, the preparation process involves mixing two polysaccharides under pulse sonication using a probe sonicator to prepare the nanoparticle. Initially, chitosan was dissolved in 1% (v / v) acetic acid solution to obtain a 1 mg/ml chitosan-acetic acid solution, which included the addition of 15mg of chitosan to a 15ml acetic acid solution. Additionally, fucoidan was dissolved in double-deionised to obtain a concentration of 1, 3 and 5 mg/ml by adding 5, 15 and 25 mg of fucoidan respectively to 5 ml of water. These solutions were used to prepare different ratios of fucoidan/chitosan nanoparticles, i.e. 1: 1, 3: 1 and 5: 1. And the loaded nanoparticles of MTX were also prepared. This included the addition of MTX equivalent of 15% (w / w) of the total amount of FC mass (0.75mg) to the polysaccharide mixture prior to the sonication step, facilitating its incorporation into the nanoparticle matrix. The polysaccharide compounds were activated with a probe sonicator for 30 seconds (3 seconds pulse on and 7 seconds pulse off). Fucoidan / Chitosan nanoparticles and Fucoidan / Chitosan nanoparticles containing MTX drug were found. The prepared drugs were centrifuged at 18,000 rpm in 20 minutes to remove all major aggregates and unwanted particles. Prepared nanoparticles are lyophilized and stored at 4°C for further analysis (Ana Isabel Barbosa et al., 2019).
Preparation of fucoidan/chitosan nanoparticle with the cross-linker
Polyelectrolyte complexes have been used to prepare marine-based nanoparticles. Various concentrations have been taken to obtain nanoparticles of smaller size and higher drug efficiency. These modified nanoparticles contain a crosslinker to reduce the size of the nanoparticle and improve the efficiency of the drug. Fucoidan/ chitosan (F/C) nanoparticles were obtained by polyelectrolyte self-assembly method with the aid of cross-linkers at room temperature. Chitosan dissolved in 1% (v / v) acetic acid to prepare a solution with 0.5 mg/ml chitosan concentration, which is 7mg of chitosan in 14ml acetic acid. Following that was the preparation of fucoidan, in which fucoidan of 0.5, 1.5 and 2.5 mg/ml of concentrate was prepared in double water containing the addition of 2.5, 7.5 and 12.5 mg respectively of fucoidan in 5 ml of water. Chitosan and Fucoidan solutions were adjusted to optimum pH. Drug nanoparticles are designed for a 1 mg/ml MTX solution. Following, various concentrations of fucoidan/chitosan nanoparticles were prepared, including 1: 1, 3: 1 and 5: 1. To produce nanoparticles loaded by MTX, add MTX (equivalent to 15% (w / w) the total value of FC) in the polysaccharide component, which facilitates its incorporation into the nanoparticle matrix, after nanoparticle production, 300 mM EDC + 120 mM NHS is added to the nanoparticle mixture. F / C solutions for the nanoparticle were stirred for 2 h at 600 rpm followed by centrifugation at 4000 rpm for 15 min and washed with ultrapure water. To avoid nanoparticle aggregation, glucose is added to the centrifugation stage. NPs were lyophilized and stored at 4°C for further analysis.
Drug entrapment efficiency
The amount of MTX entrapped at different concentrations was found by calculating the percentage of drug trapped inside the nanoparticles. Nanoparticles with and without a cross-linker were centrifuged at 20,000 rpm for 30 minutes to extract the compound. 50 uL of each formulation was dissolved in 2 ml of DMSO and then centrifuged at 8000 rpm for 15 minutes to extract polysaccharides. The centrifugal formation was measured by the UV-vis spectrophotometer at 303nm (Wu et al., 2016).
% EE
Characterization
Dynamic light scattering (DLS)
The Dynamic Light Scatter (DLS) method is most widely used to quantify particle size within the nanometer scale. The sample is illuminated by a laser beam and the variations of the scattered light are detected by a fast photon detector at the known scattering angle θ. Simple DLS instruments that measure at a fixed angle will calculate the average particle size over a restricted range of sizes. Eppendorf's were centrifuged twice at 5000 rpm for 10 minutes. The supernatant was discarded and resuspended in 1ml of water. Followed by the transfer of 900 μl of water and 100 μl of the solution to the new Eppendorf, the particle size and volume distribution of the generated compound were determined using the Zetasizer Nano ZS DLS technique. The quantitative analysis lasted 180's and was conducted at 25°C. Zeta potential measurements were carried out by dip cells in automatic mode. DLS analysis was also performed for samples such as fucoidan/chitosan nanoparticle and fucoidan/chitosan nanoparticle loaded with MTX (Deepika et al., 2019).
Fourier Transforms Infrared Spectroscopy (FTIR)
Fourier transform infrared spectroscopy is an analytical method used to identify organic, polymeric and certain inorganic materials. This technique tests infrared radiation absorption by the sample material and wavelength. Infrared absorption bands are used to classify molecular components and structures. The nanoparticles were separated by centrifugation for 10 min at 5000 rpm for FTIR measurements. The supernatants were discarded and resuspended with 250ul water. Subsequently, they were collected and deeply frozen for 72 h and lyophilized. FT-IR spectral analysis using the Gary 660 FT-IR spectrometer at the wavelength range 400-4000 / cm This analysis was performed for various models such as MTX drug, fucoidan/chitosan nanoparticle and fucoidan/chitosan nanoparticle loaded with MTX drug (Deepika et al., 2019).
Scanning electron microscopy (SEM) analysis
Scanning Electron Microscope (SEM) is a type of electron microscope that produces a sample image by scanning the surface with a focused electron beam. The electrons interact with the atoms in the sample, forming various signals containing information about the surface area and composition of the sample. Samples were placed overnight in a deep freezer at 20°C and then lyophilized. SEM was conducted on the FEI Quanta FEG 200-high resolution electron scanning microscope within a wavelength of 5 to 200 µm (Oliveira, Neves, Reis, Martins, & Silva, 2018).
Results and Discussion
Dynamic Light Scattering
Dynamic Light Scattering is a method that relies on light and its interactions with different sized particles. This method is used to determine the distribution of small particle sizes, particularly in the range of 2–500nm (Tomaszewska et al., 2013).
Nanoparticle Formulation (F/C Ratio) |
Size (nm) |
Zeta potential (mV) |
---|---|---|
Without Cross linker |
||
1:1 -MTX |
304.3 |
-0.2 |
3:1 -MTX |
220.7 |
-0.1 |
5:1 -MTX |
296 |
-0.0 |
1:1 +MTX |
786.2 |
-0.0 |
3:1 +MTX |
254.6 |
+0.4 |
5:1 +MTX |
691 |
-0.5 |
With Cross linker |
||
1:1 -MTX |
6.2 |
-0.1 |
3:1 -MTX |
3.8 |
-0.1 |
5:1 -MTX |
0.4 |
-0.0 |
1:1 +MTX |
39.3 |
-0.1 |
3:1 +MTX |
210.1 |
-0.1 |
5:1 +MTX |
441.5 |
-0.0 |
Nanoparticle (F:C) |
Entrapment Efficiency (%) |
---|---|
Without Crosslinker |
|
1:1 |
37.3 |
3:1 |
26.7 |
5:1 |
64 |
With Crosslinker |
|
1:1 |
11.7 |
3:1 |
35 |
5:1 |
41.7 |
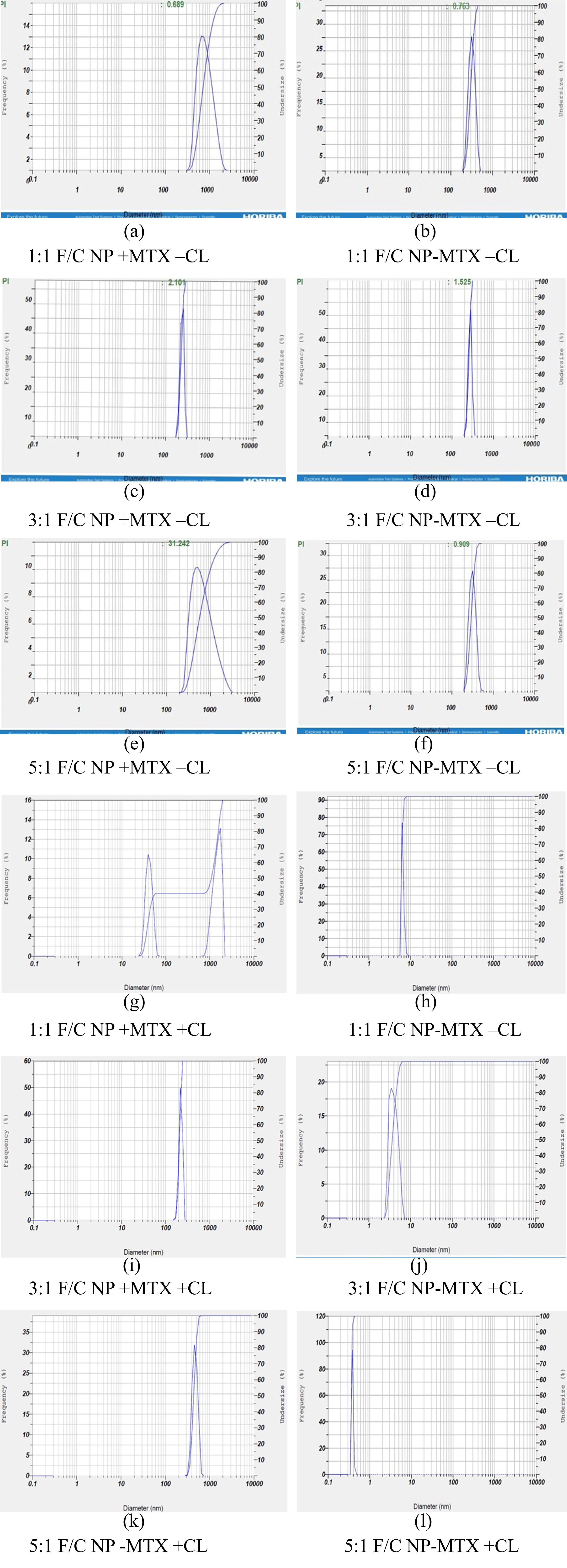
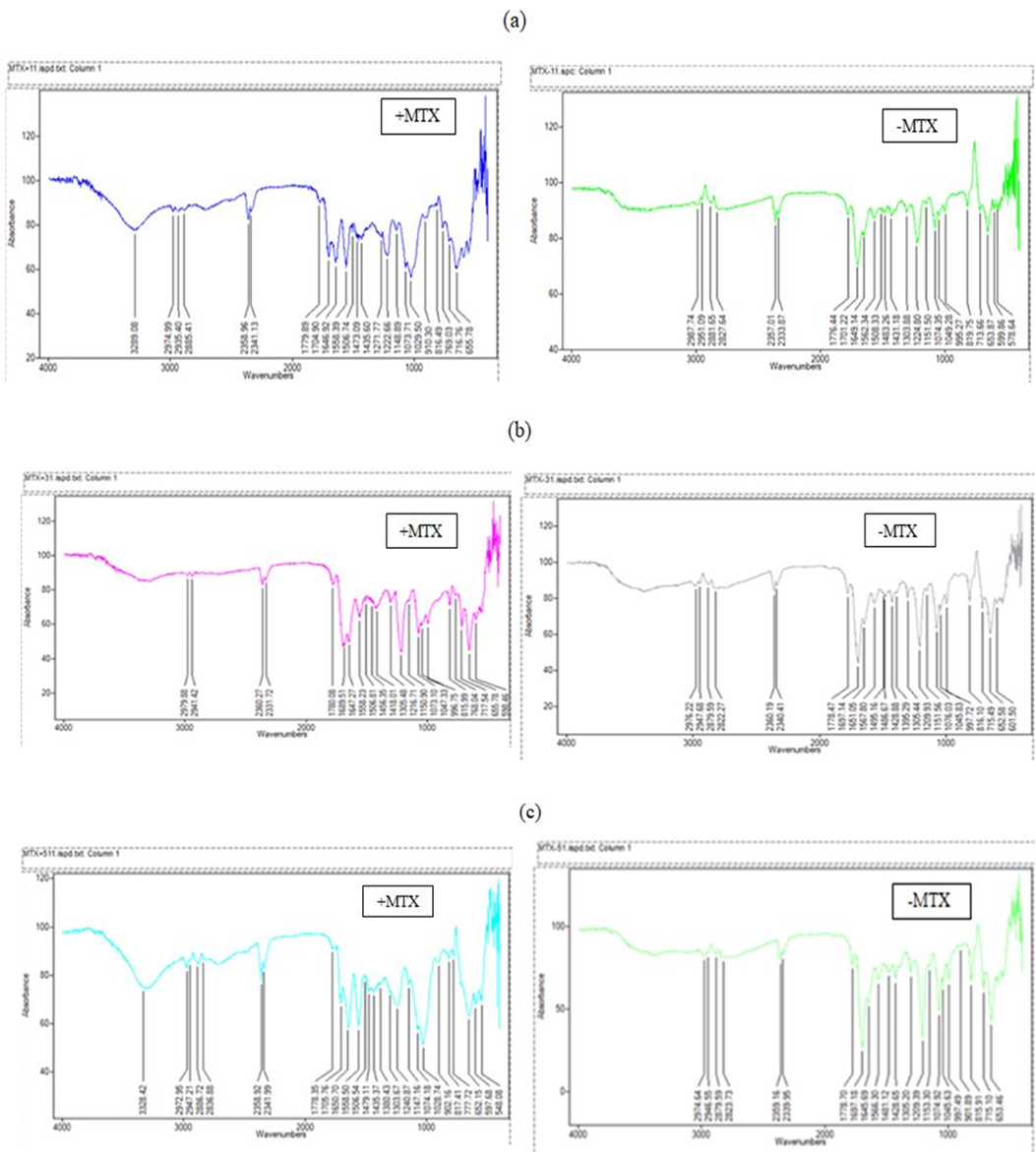
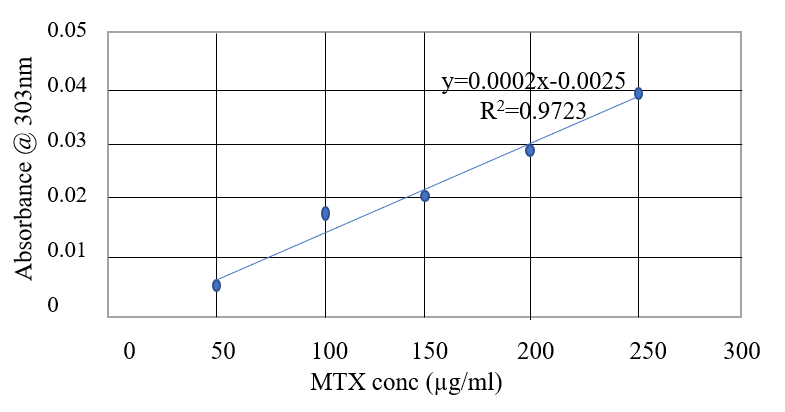
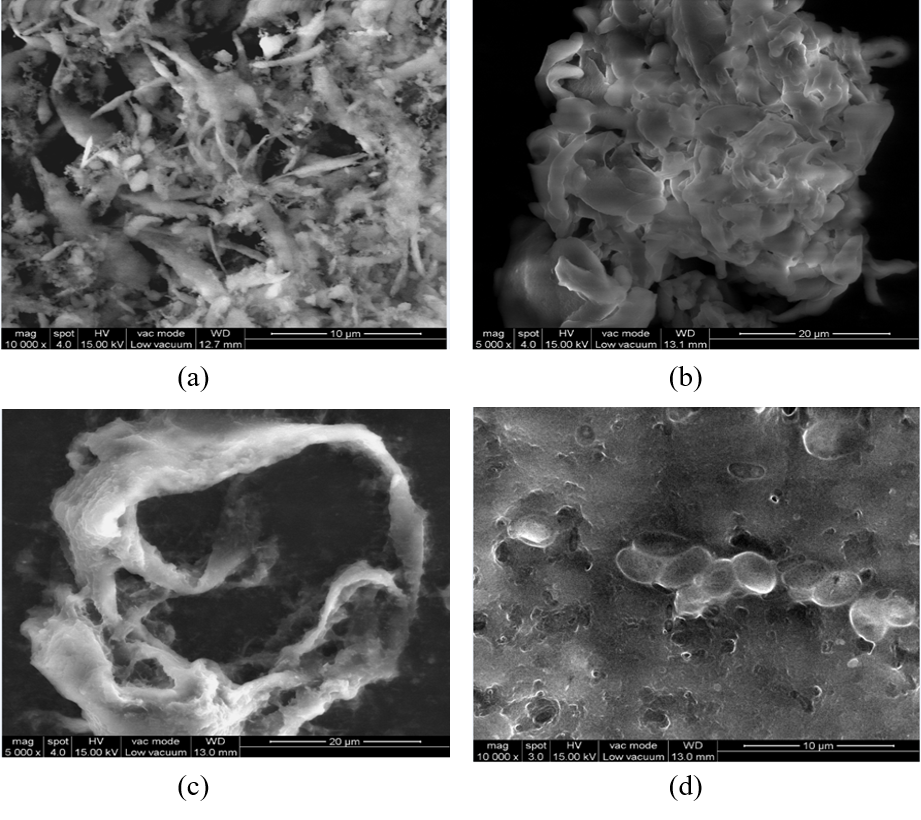
The Nanoparticle formulations were made using three different Fucoidan(F)/Chitosan(C) ratios; 1F/1C, 3F/1C and 5F/1C, was previously performed for anti-inflammatory activity and quercetin delivery (Coutinho et al., 2019). The nanoparticle formulations were prepared using two different methods, synthesized with and without cross-linkers (EDC and NHS). Both these samples were characterized through DLS analysis to determine the changes in size and zeta potential in the nanoparticles, which the use of cross-linkers would have incurred. Testing was done for nanoparticles prepared with the inclusion and exclusion of methotrexate. The nanoparticle sizes were seen to have a wider range of sizes but within the range of the various studies previously done using nanoparticles in these formulations (Barbosa et al., 2019; Oliveira et al., 2018).
Based on the results obtained in Figure 1 (a-l), it was determined that the use of the 3:1 and 5:1 F/C formulations is ideal size nanoparticles. However, it was seen that the nanoparticles could be deemed relatively large for use without the addition of the crosslinker. As depicted in Table 1, the formulation 3:1 & 5:1 F/C nanoparticles, which contained the MTX drug but not the cross-linkers with average size 254.6 nm and 691 nm, respectively. This size decreased considerably with the addition of the aforementioned cross-linkers. The 3:1 F/C nanoparticles were seen to be 210.1 nm, while the 5:1 F/C nanoparticles averaged at 441.5 nm, the difference being considerably larger in the 5:1 formulation. This veritable reduction was anticipated from the already available data, which depicted that fucoidan/chitosan nanoparticles prepared in a similar manner with a similarly sized drug (such as Gemcitabine) have a reduced size. The average calculated size with the DLS method is 141 nm (Oliveira et al., 2018). Moreover, from the previous studies, the particle size and polydispersity index of the fucoidan-coated AgNPs and chitosan–fucoidan complex-coated AgNPs obtained were seen to be 53.19 ± 2.23 nm and 73.09 ± 9.54 nm, respectively (Venkatesan, Singh, Anil, Kim, & Shim, 2018). The difference in the concentrations of both fucoidan and chitosan used to create the nanoparticle has led to the larger nanoparticle size.
FTIR - Fourier transform infrared spectroscopy
FTIR is used to identify the presence of different functional groups by calculating the vibrational frequencies of the chemical bonds between the atoms. We tested the various nanoparticles formed to visualize these bonds as well as to confirm the action of EDC and NHS in the nanoparticles with the crosslinkers.EDC interacts with carboxyl groups and forms the amine-reactive O-acylisourea intermediate, which respond directly to basic amines to form an amide bond and isourea product. The necessity of quenching the EDC activation is the reason why NHS is also added, as the coupling of the EDC with NHS will form an NHS ester more stable than the O-acylisourea intermediate and allows for efficient conjugation to primary amines at physiologic pH (Oliveira et al., 2018; Park, Vo, Kang, Oh, & Lee, 2015). The FTIR analysis was done to determine the changes which the addition of EDC and NHS would do to the nanoparticles. For the nanoparticles obtained with the crosslinkers, the data was obtained and then analyzed using the Essential FTIR software.
Previously documented studies on the preparation of nanoparticles showed that the linking of Fucoidan and Chitosan without crosslinkers depicted characteristic peaks of both Fucoidan and Chitosan individually, although a shift in the C=O amide group was visible, which corresponded to the same group being present in the Chitosan spectrum, the change in environments making the shift in the nanoparticle possible (Lee & Lim, 2014).
Another phenomenon found in other studies depicted that the addition of conjugates in the preparation of fucoidan/chitosan nanoparticles (e.g. taurine, genipin) led to similar significant shifts in the spectra produced, thus depicting the conjugate/crosslinker action through FTIR studies (Lin et al., 2017; Wu, Don, Lin, & Mi, 2014). Therefore, it became essential to confirm and assess the EDC/NHS action during the creation of the crosslinked nanoparticles, especially as the few experiments which involved these crosslinkers with this particular nanoparticle formulation did not study this characteristic (Oliveira et al., 2018).
From experiments conducted on nanoparticles created without crosslinkers, we could identify N-H stretching in the 3000-3500 cm-1 range for these specific nanoparticles. This stretch is absent in most of the nanoparticles which use EDC and NHS during preparation, suggesting that the N-H groups have been utilized in forming new bonds. Also seen in the 2800-3000 cm-1 region is an increase in C-H stretching in the nanoparticles with crosslinkers from Figure 2 (a)-(c).
The graphs given below were compared to the ones obtained from nanoparticles that do not contain the specified cross-linkers, thus enabling us to detect the action of the crosslinkers. The presence of the peaks representing C=O (1732, 1742) aldehyde/carboxyl grp was seen in graphs for the nanoparticles not using the cross-linkers (and methotrexate). These particular peaks not visible in any of the graphs for nanoparticles prepared using the crosslinkers. Instead, peaks in the 1690-1710 range for primary amide group C=O stretch were visible as the EDC and NHS reactions proceeded to create the amide groups in these nanoparticles.
Drug Entrapment Efficiency
The entrapment efficiency of three different formulations was calculated for nanoparticles with and without the crosslinkers. This was done by using UV/VIS spectroscopy to determine the concentration of the non-entrapped drug and then using the formula to determine the entrapment efficiency of the different nanoparticles. Based on the obtained result Figure 3, we concluded that the 5:1 F/C nanoparticle would be ideal for use due to the relative high drug entrapment efficiency as seen in Table 2, with efficiencies of 64% and 41.7% for nanoparticles created with and without the use of crosslinkers respectively. It was also observed that the entrapped drug is slowly released for 24 hr and then maintained a steady profile for the next 72 hrs (Oliveira et al., 2018). Thus, 3/1 and 5/1 fucoidan/chitosan ratios produced nanoparticles that are capable of delivering seized drugs, which can be used as a cancer treatment. Well-charged CFNs are adjusted because the relative charge concentration of amino groups loaded into CFNs was greater than that of negative ion sulphates loaded in CFNs (Lee & Lim, 2016). The entrapment efficiencies for the nanoparticles, including the crosslinkers, were greater than anticipated from the available literature.
SEM – Scanning Electron Microscopy
SEM is an integrated tool used to determine the size, shape and surface of a nanoparticle. It is was performed by FEI Quanta FEG 200-High resolution scanning electron microscope within a region of 5 to 200 µm. SEM was performed for Fucoidan/chitosan nanoparticle with and without crosslinker for the proportion 5:1F/C. Without the cross-linker and the drug MTX, fucoidan/chitosan nanoparticles showed bond formation, uniform circles and bunches Figure 4 (a). The particles form three-dimensional clusters of fucoidan/ chitosan nanoparticle along with the entrapment drug MTX Figure 4 (b). In the presence of the cross-linker and drug, the nanoparticles form clusters and small shaped spherical structures along with the entrapped drug Figure 4 (d). In the presence of the crosslinker, the particle forms a hollow spherical shape structure. The size of the particle reduces, and no entrapment of the drug is observed in Figure 4 (c). The addition of a cross-linker allows for the effective release of the drug from the cells and reduces the nanoparticle size that has been detected and confirmed by DLS analysis.
SEM examination is carried to elucidate the morphological properties of nanoparticles. Studies propose that Gemcitabine stacked F/C nanoparticle was found to be round in shape and size smaller than 100nm in the microscopic analysis (Oliveira et al., 2018). The morphology of Methotrexate nanoparticle (MTX-NPs), Curcumin nanoparticle (CUR-NPs), and MTX-CUR-NPs-8 were studied as spherical with a slight aggregation, especially in CUR-NPs (Vakilinezhad et al., 2019). In the case of methotrexate loaded with chitosan nanoparticles, the average dimension ranges from 120-360nm (Ekinci et al., 2015). Usually, SEM-analyzed nanoparticles are aggregated by several dozen smaller particles, irregular in form and differ in mean dimension. Other particles such as FUC / TMC / TPP-MP incorporate easily simplified content due to the strong interaction of the positive charge of TMC and the negative charge of TPP and FUC (Hayashi, Sasatsu, Machida, & Onishi, 2011). SEM is also used to analyse the surface morphology, pore distribution and pore size in studies related to bone tissue engineering. Previous studies of Chi-Alg-fucoidan and TCP-Ch-Fucoidan prove that high pore size enables scaffolds to allow cell adhesion, proliferation and nutrient supply, which will emphasize the proper growth of bone tissue (Puvaneswary et al., 2015).
Conclusion
In this study, the fucoidan/chitosan nanoparticles were prepared based on their electrical interactions and classified as optimal for methotrexate topical distribution. Cross-linked nanoparticles are quite stable for seven days. However, after three days, NPs without cross-linker begin to increase in size, indicating that they begin to decompose at physiological osmosis. Cross-linkers reduce the size of nanoparticles and increase the effectiveness of drug release. The 5F / 1C nanoparticles showed a significant decrease in size from 691nm (without cross-linker) to 441nm compared to other ratios, recording the highest entrapment efficiency. Therefore this formula can be further analyzed for its bioavailability, efficacy, efficiency and anticancer activity against suspension cell line using MTT, cell cycle analysis and apoptosis assay.